|
Indian Pediatr 2018;55: 865-870 |
 |
Postnatal Maturation of Amplitude Integrated
Electroencephalography (aEEG) in Preterm Small for Gestational
Age Neonates
|
Kamaldeep Arora,
Anu Thukral, M Jeeva Sankar, Sheffali Gulati, Ashok K
Deorari, Vinod K Paul and Ramesh Agarwal
From Newborn Health Knowledge Centre, WHO
Collaborating Centre For Training and Research in Neonatal Care,
Department of Pediatrics, All India Institute of Medical Sciences, New
Delhi, India.
Correspondence to: Dr Ramesh Agarwal, Professor,
Division of Neonatology, Department of Pediatrics, All India Institute
of Medical Sciences, New Delhi, India.
Email: [email protected]
Received:January 19, 2017;
Initial review: January 20, 2017;
Accepted: July 21, 2018.
|
The primary objective was to evaluate
the postnatal maturation pattern on aEEG during first two weeks of life
in clinically stable and neurologically normal preterm small for
gestational age (PSGA) and gestation matched (1 week) preterm
appropriate for gestational age (PAGA) neonates born between 300/7 and
346/7 weeks of gestation. Methods: Serial aEEG tracings were
recorded on 3rd, 7th and 14th day of life. The primary outcome was total
aEEG maturation score. Three blinded assessors assigned the scores.
Results: We analyzed a total of 117 aEEG recordings in 40 (19 PSGA
and 21 PAGA) neonates. The baseline characteristics were comparable
except for birthweight [1186 (263) vs 1666 (230) g]. There was no
difference in the mean (SD) total scores on day 3 (9.0 (1.8) vs.
9.5 (1.1), P=0.32) and day 14 of life, but was lower in PSGA
infants on day 7 (8.6 (2.4) vs. 10.1 (1.1), P=0.02). On
multivariate analysis, maturation of PSGA neonates was found to be
significantly delayed at any point of life from day 3 to day 14 (mean
difference, -0.8, 95 % CI: -1.6 to -0.02, P=0.04). Conclusion:
Lower aEEG maturation score on day 7 possibly indicates delayed
maturation in PSGA neonates in the first week of life.
Keywords: Electrophysiology, Neuronal
maturation, Outcome.
|
P reterm neonates are at a risk of developmental
delay, visual and hearing problems, behavioral problems and learning
disabilities [1]. Nearly 20% of the preterm neonates are growth retarded
thus further increasing their jeopardy [2]. Preterm small for
gestational age (PSGA) neonates have decreased cortical volume, myelin
reduction, neuronal degeneration in the hippocampus and axonal
degeneration in periventricular region [2,3].
The brain electrographic pattern and activity
assessed by electroencephalogram (EEG) is an indicator of neuronal
activity and differentiation [4].
Amplitude integrated electroencephalogram (aEEG) is
used as a bedside device for continuously assessing the cerebral
electrical activity and its practical and prognostic use has been
demonstrated in many studies in term and preterm infants [5-11]. We
hypothesized that postnatal maturation of aEEG would be delayed in PSGA
as compared to their AGA counterparts. To date, only three studies from
developed countries have assessed the aEEG maturation specifically in
PSGA neonates [12-14]. The evidence is thus limited, with aspect to the
aEEG maturation pattern in stable preterm neonates which may be
important to decipher, given the alteration of aEEG patterns by
different morbidities and intracranial insults [15]. Hence, we designed
this study to evaluate the aEEG maturation pattern in preterm small for
gestational age neonates and compare their maturation with appropriate
for gestational age neonates, by using a validated aEEG maturation
scoring.
Methods
This prospective observational study was conducted in
the neonatal intensive unit of our institution from June 2010 to
December 2011. Written informed consent was obtained from one of the
parents, and the study was approved by the Institutional ethics
committee of All India Institute of Medical sciences, New Delhi, India.
Clinically stable preterm neonates (30 +0/7
to 34+6/7 weeks) were
included. Neonates with perinatal insult (Apgar score 2 or less at 5
minutes), major congenital anomalies, any grade of intraventricular
hemorrhage (IVH), periventricular leucomalacia (PVL), necrotizing
enterocolitis, evidence of central nervous system infection, clinical
seizures or suspected metabolic disorders
were excluded.
A log book was maintained to keep track of all the
neonates. Best gestational age (GA) was assigned based on the last
menstrual period, or the modified Expanded New Ballard score, if the
mother was not sure about the dates or if the discrepancy in assessment
and dates was more than two weeks [22]. SGA was defined as less than 10 th
centile for gestation according to the AIIMS intrauterine charts [23].
Cranial ultrasound (CUS) was performed on all
subjects by the radiologist, as per unit protocol at postnatal age 3, 7
and 14 day of life to rule out intraventricular hemorrhage (IVH),
periventricular leucomlacia (PVL) or any cranial malformation.
The aEEG tracings were recorded using the amplitude
integrated EEG machine (Nicolet, Viasys, San Diego CA, United States),
as per standard methodology [24]. Each recording was done for at least
4-hour duration to ensure good sleep-wake cycling recording [25].
Continuous impedance check was done throughout the recording. The
tracings with impedance >20 kOhm were discarded.
The primary outcome variable was total aEEG
maturation score as per Burdjalov, et al. [7]. Each tracing of
the subject was analyzed by three independent assessors. The methods
adopted for scoring the aEEG traces are summarized in Box I.
The secondary outcome variables were the scores of the four individual
parameters of maturation.
Box I Methods Adopted for Assigning The
aEEG Score
Step 1: Training of assessors
Three independent assessors* were trained for
analyzing the recorded aEEG’s. Each assessor was provided with
study material and video CD of aEEG. After the training
sessions, assessors were provided with sample aEEG records, of
various patients with varying gestational age and relevant
clinical scenarios.
Step 2: Analyzing the aEEG recordings
The assessors were blinded to gestation,
birth weight, and postnatal age at which aEEG was recorded. They
analyzed the aEEG tracings individually and assigned the scores
to each component of aEEG trace. They submitted their scores to
the principal investigator (KA).
Step 3: Assigning the final scores
The principal investigator assigned the final
scores based on the concordance between the three outcome
assessors – if the scores given by all three assessors were the
same, that score was recorded as the final score. However, if it
was different, the three assessors reviewed the aEEG tracing
together to reach a consensus score. This score was then
recorded as the final score. Concordance among assessors was
>95%.
*The outcome assessment team (three members)
included neonatology fellows/faculty level personnel with
experience and competency in neonatology.
|
According to the study done by Burdjalov, et al.
[7] the maturation score in neonates with 30-34 weeks of gestation on
day 3 of life was 10. A study on conventional EEG in term SGA neonates
versus the term AGA neonates followed till three months of age revealed
50% less amplitude in SGA cohort as compared to AGA cohort because there
was no study on mean total score (summation of all four components of
the aEEG tracing) evaluation in preterm infants prior to the initiation
of this study we assumed the difference in mean total score to be the
same as the score in amplitude alone in conventional EEG study. To
observe the difference in mean total score of at least 50% among the two
cohorts, with alpha error 5% and power of study 80 %, we needed to
enroll 20 neonates in each group.
Statistical analysis: The data were analyzed
using Stata version 11 (Stata Corp, College Station, TX). Normally
distributed, continuous data was analyzed by Student t test and
data which was non-normally distributed was analyzed by Wilcoxon rank
sum test. Chi-square or Fisher exact test were used to analyze
categorical data. Generalized estimating equation (GEE) analysis was
used to analyze the change in score from first aEEG recording to the
final one after adjusting for correlated values. P value of <0.05 was
considered as significant.
Results
Of the total 159 neonates assessed for eligibility,
119 neonates were excluded (Fig. 1). The maternal
characteristics and baseline neonatal characteristics in PSGA (n=19)
and PAGA (n=21) cohorts were similar in the two groups (Table
I). No neonate in either group developed retinopathy of prematurity
(ROP) requiring treatment, or patent ductus arteriosus (PDA) needing any
intervention. There was no death in either cohort during the study
period.
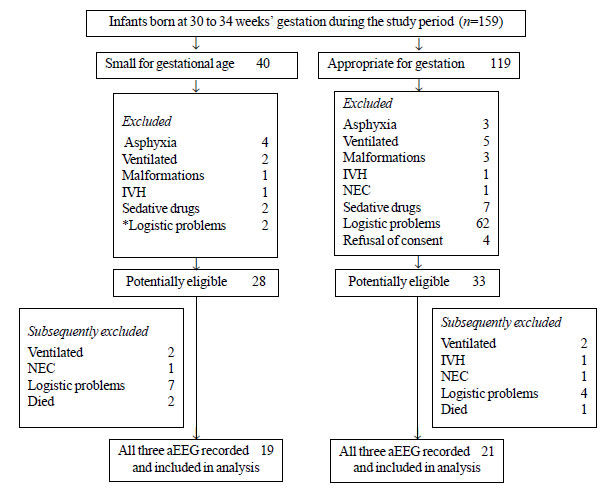 |
Fig. 1 Flow of Patients in the study.
|
TABLE I Baseline Variables of the Study Population
Variable |
SGA (n=19) |
AGA (n=21) |
Maternal characteristics |
Age (y)*
|
27.8 (3.3) |
26.8 (3.9) |
Multigravida#
|
10 (52) |
16 (76) |
Booked#
|
16 (84) |
21 (100)
|
Gestational hypertension# |
4 (21) |
2 (9.5) |
Gestational diabetes |
3 (15.7) |
3 (14.2) |
PPROM# |
1 (5.2) |
2 (9.5)
|
Neonatal characteristics: At birth |
Gestation (wk)$ |
32 (30-34) |
33 (30-34) |
Weight (g)* |
1186 (263) |
1666 (230) |
Male# |
8 (32) |
15 (60) |
Mode of delivery# |
|
|
Vaginal delivery |
7 (37) |
12 (57) |
Twins# |
7 (36.8) |
9 (43) |
Apgar at 5 min$ |
8 (7-9) |
8 (6-9) |
Need for oxygen# |
2 (10.5) |
3 (14.2) |
BMV# |
2 (10.5) |
0 |
Postnatal |
Sepsis# |
6 (31) |
4 (19) |
NEC (I)# |
2 (10.5) |
0 |
Transient tachypnea of newborn# |
7 (36.8) |
9 (42.8) |
Respiratory distress syndrome |
2 (10.5) |
2 (9.5) |
CPAP# |
5 (26.3) |
3 (14.2) |
Total parenteral nutrition# |
6 (31.5) |
1 (4.7) |
Bronchopulmonary dysplasia# |
1 (5.2) |
0 |
VH (I)# |
1 (5.2) |
0 |
Data presented as *mean (SD), $median (range), #number
(%); PPROM: Preterm premature rupture of membranes; BMV: Bag and
mask ventilation. |
There was no difference in the total scores of the
two cohorts on day 3 of life and day 14 (Table II).
However, PSGA neonates had lower total score on day 7. On multivariate
analysis, maturation score of PSGA neonates was found to be
significantly delayed at any point of life from day 3 till day 14 of
life (mean difference, -0.8, 95 % CI: -1.6 to -0.017, P=0.045).
TABLE II Amplitude-integrated EEG Total Score Among Small for Gestational Age (SGA) and
Appropriate for Gestational Age (AGA) Neonates
Day of Life |
SGA (n=19) |
AGA (n=21) |
Mean difference (95 % CI)# |
3 |
9.0 (1.8 ) |
9.5(1.1) |
-0.5 (-1.5 to 0.5) |
7 |
8.6 (2.4) |
10.1(1.1) |
-1.4 (-2.6 to -0.3)$ |
14 |
10.2 (1.3 ) |
10.7 (0.8) |
-0.5 (-1.2 to 0) |
Values expressed as mean (SD); #Adjusted for
correlated values by generalized estimating equation (GEE);
$P=0.02. |
There was no difference in the Continuity scores of
the two cohorts at any time point of assessment (Table III).
The Cycling score and Lower border score were similar in the two groups
on day 3 and day 14. PSGA neonates had lower SWC score and ‘Lower border
score’ on day 7. The bandwidth score in PSGA neonates was lower than the
PAGA cohort on day 14 (3.3 (0.6 vs.3.7 (0.4, 95% CI 0 to 0.7).
TABLE III aEEG Score (Cycling, Continuity, Lower Border, and Bandwidth) Among Small for Gestational Age (SGA)
and Appropriate for Gestational Age (AGA) Neonates (N=40)
aEEG |
SGA* |
AGA* |
Mean difference |
P |
recording |
(n=19) |
(n=21) |
(95 % CI)# |
value |
aEEG continuity score |
Day 3 |
1.7 (0.4) |
1.9 (0.3) |
-0.1 (-0.36 to 0) |
0.31 |
Day 7 |
1.7 (0.5) |
2.0 |
-0.2 (-0.4 to 0) |
0.08 |
Day 14 |
2.0 |
2.0 |
-0.5 (-1.2 to 0) |
0.11 |
aEEG cycling score |
Day 3 |
2.2 (0.8) |
2.4 (0.7) |
-0.1 (-0.6 to -0.3) |
0.61 |
Day 7 |
2.1 (1.1) |
2.7 (0.7) |
-0.6 (-1.2 to 0) |
0.03 |
Day 14 |
2.8 (0.8) |
3.0 (0.6) |
-0.2 (-0.6 to 0) |
0.39 |
aEEG lower border score |
Day 3 |
1.8 (0.8) |
2.0 |
-0.1 (-0.2 to 0) |
0.13 |
Day 7 |
1.7 (0.4) |
2.0 |
-0.2 (-0.4 to 0) |
0.02 |
Day 14 |
2.0 |
2.0 |
- |
- |
aEEG bandwidth score
|
Day 3 |
3.1 (0.6) |
3.3 (0.4) |
-0.1 (-0.5 to 0) |
0.60 |
Day 7 |
3.0 (0.8) |
3.4 (0.5) |
-0.4 (-0.8 to 0) |
0.05 |
Day 14 |
3.3 (0.6) |
3.7 (0.4) |
-0.3 (-0.7 to 0) |
0.03 |
*Values expressed as mean (SD); #Adjusted for
correlated values by generalized estimating equation (GEE). |
Discussion
This study evaluates the maturation pattern of aEEG
in clinically stable PSGA neonates neonates during the first two weeks
of life. This study evaluated both prematurity and the SGA status on
maturation pattern using aEEG. PSGA neonates had similar total scores as
PAGA neonates on day 3 of life and day 14 and a lower total score on day
7. The maturation score of PSGA neonates in the present study was found
to be significantly delayed at any point of life from day 3 till day 14
of life.
The plausible explanation of the delayed maturation
score at any time point for PSGA neonates seems to be related to the
underlying reasons for growth retardation. The phenomenon for delay at
day 7 but not at day 3 or day 14 has not been understood very clearly.
The rationale behind this may be the delay in starting the enteral
feeds, which may show delay at day 7. Till date no studies have looked
at the long term outcome of this transient maturity. Malnutrition during
the critical phase of brain development may translate into delayed
myelination and subsequently to delayed maturation pattern [3]. A recent
study reported similar aEEG total scores in PAGA and PSGA neonates [12].
This study, however, did not specifically comment on the maturation
delay at any time point but revealed a higher number of bursts per hour
(objective quantitative parameter which indicates slower postnatal
adaptation of electrocortical function in PSGA neonates); thus
indirectly indicating a delay in electrocortical activity.
Nearly all PSGA neonates in the present study
achieved continuous pattern on aEEG at enrollment. These results are
similar to previous studies [12,14]. Continuity is one of the earliest
parameters to be achieved in aEEG recording [7,8]. It represents the
general status of the brain [26].
Our neonates were stable and had no clinical evidence of
neurological dysfunction, so the attainment of a continuous pattern on
aEEG is not unexpected at 30-34 weeks.
Our study suggested a comparable cycling pattern in
PSGA vs. PAGA neonates except on day 7 (second recording). The
study by Feler, et al. [13] suggested a comparable cycling
pattern in the two groups while the study by Schwindt, et al.
[14] suggested less cycling
activity in the SGA neonates. It may be difficult to compare our results
with the findings of the latter two studies as both these studies
evaluated the SWC pattern at a single time point in the first two weeks
which may not be representative of the changes which occur with
increasing postnatal age. SWC represents the integration of functions of
the higher centers of the brain and a premature sleep wake cycling in
PSGA neonates may represent a delay in synaptogenesis of the neurons
[22,26]. The study by Feler, et al. [13] also reported a higher
aEEG bandwidth in the PSGA neonates. The bandwidth decreases and the
lower border elevates with increasing maturity [7,13].
The strengths of our study include the evaluation of
clinically stable preterm neonates without any major morbidities
or requiring sedation [13,23,25]. In addition, low
impedance during the recording was ensured by adequate electrode
contact, continuous check on impedance and uninterrupted study periods
[26]. The duration of each tracing was atleast 4 hours. This helped
increase the chance of detecting even immature sleep-wake cycles.
In addition, the serial recordings on neonates
gave an insight in the maturation pattern at different time points,
rather than one study time point [22]. We used the controls from the
same population rather than other reference standards. The aEEG tracings
were assessed by three blinded assessors.
Our study had a few limitations, including a small
sample size. It is difficult to enroll stable PSGA neonates because this
subgroup of neonates suffers from high risk of perinatal asphyxia,
necrotizing enterocolitis and requirement of mechanical ventilation. The
second limitation was a short follow up aEEG recordings of these
neonates. In addition, ongoing recordings continuing upto term gestation
may have helped us to delineate the specific maturation pattern in these
neonates.
The maturation score of clinically stable and
neurologically normal P-SGA neonates PSGA neonates was found to be
significantly delayed at any point of life from day 3 till day 14 of
life. Further studies evaluating progressive aEEG maturation till term
age and the impact of the maturation pattern on subsequent
neuro-developmental outcomes may provide further insight into the
developmental pattern in these neonates.
Contributors: KA: primary responsibility for
protocol development, study implementation, data management and writing
the manuscript; AT, JS: development of the protocol and supervised
implementation of the study, analysed the tracings, assigned the scores
and contributed to writing of the manuscript. JS: helped in the
statistical analysis. VKP, AKD, SG: protocol development, gave critical
inputs in designing and execution of the study, data analysis and in
manuscript writing. RA: conceptualized and designed the study, analyzed
the aEEG tracings and assigned the scores, finalized the manuscript. He
will act as guarantor of paper. All authors have reviewed the final
manuscript and approved it.
Funding: None; Competing Interest:
None stated.
What This Study Adds?
•
Preterm SGA neonates show similar
total aEEG maturation score as Preterm AGA neonates on day 3 and
14, but a lower score on day 7 possibly indicating a delayed
maturation in these neonates in the first week of life.
|
References
1. Ward RM, Beachy JC. Neonatal complications
following preterm birth. BJOG. 2003;110S:8-16.
2. Vanderveen JA, Bassler D, Robertson CMT, Kirpalani
H. Early interventions involving parents to improve neurodevelopmental
outcomes of premature infants: a meta-analysis. J Perinatol.
2009;29:343-51.
3. Ozdemir OMA, Ergin H, Sahiner, T.
Electrophysiological assessment of the brain function in term SGA
infants. Brain Res. 2009;1270:33-8.
4. Morgane PJ, Austin K, Siok C, LaFrance R, Bronzino
JD. Power spectral analysis of hippocampal and cortical EEG activity
following severe prenatal protein malnutrition in the rat. Brain
Res.1985;354:211-8.
5. Toet MC, van der Meij W, de Vries LS, Uiterwaal
CS, van Huffelen KC. Comparison between simultaneously recorded
amplitude integrated electroencephalogram (cerebral function monitor)
and standard electro-encephalogram in neonates. Pediatrics.
2002;109:772-9.
6. Clancy RR, Dicker L, Cho S, Cook N, Nicolson SC,
Wernovsky G, et al. Agreement between long-term neonatal
background classification by conventional and amplitude-integrated EEG.
J Clin Neurophysiol.2011; 28:1-9.
7. Burdjalov VF, Baumgart S, Spitzer AR. Cerebral
function monitoring: A new scoring system for the evaluation of brain
maturation in neonates. Pediatrics. 2003;112:855-61.
8. Thornberg E, Thiringer K. Normal pattern of the
cerebral function monitor trace in term and preterm neonates. Acta
Paediatr Scand.1990;79:20-5.
9. Olischar M, Klebermass K, Kuhle S, Hulek M, Kohlhauser
C, Rücklinger E, et al. Reference values for amplitude-integrated
electroencephalographic activity in preterm neonates younger than 30
weeks’ gestational age. Pediatrics. 2004;113:e61-6.
10. Soubasi V, Mitsakis K, Nakas CT, Petridou S, Sarafidis
K, Griva M, et al. The influence of extrauterine life on the aEEG
maturation in normal preterm neonates. Early Hum Dev. 2009;85:761-5.
11. Toet MC, Hellström-Westas L, Groenendaal F, Eken
P, de Vries LS. Amplitude integrated EEG 3 and 6 hours after birth in
full term neonates with hypoxic-ischaemic encephalopathy. Arch Dis Child
Fetal Neonatal Ed. 1999;81:F19-23.
12. Griesmaier E, Burger C, Ralser E, Neubauer V, Kiechl-Kohlendorfer
U. Amplitude- integrated electroencephalo-graphy shows mild delays in
electrocortical activity in preterm neonates born small for gestational
age. Acta Paediatr. 2015;104:e283-8.
13. Yerushalmy-Feler A, Marom R, Peylan T, Korn A,
Haham A, Mandel D, et al. Electroencephalographic characteristics
in preterm infants born with intrauterine growth restriction. J Pediatr.
2014;164:756-61.
14. Schwindt E, Thaller C, Czaba-Hnizdo C, Giordano
V, Olischar M, Waldhoer T, et al. Being born small for
gestational age influences Amplitude-integrated electroencephalography
and later outcome in preterm infants. Neonatology. 2015;108:81-7.
15. Sommers R, Tucker R, Harini C, Laptook AR.
Neurological maturation of late preterm infants at 34 week assessed by
amplitude integrated electroencephalogram. Pediatr Res. 2013;74:705-11.
16. Ballard JL. Khoury JC, Wedig K, Wang L, Eilers-Walsman
BL, Lipp R. New Ballard Score: Expanded to include extremely premature
infants J Pediatr. 1991;119:417-23.
17. Singh M, Giri SK, Ramachandran K. Intrauterine
growth curves of live born single babies. Indian Pediatr. 1974;11:475-9.
18. Kidokoro H, Inder T, Okumura A, Watanabe K. What
does cyclicity on amplitude-integrated EEG mean? J Perinatol.
2012;32:565-9.
19. Lena Hellström-Westas. An Atlas of
Amplitude-Integrated EEGs in the Newborn, Second Edition: Informa
healthcare; 2008.
20. Kidokoro H, Kubota T, Hayashi N, Hayakawa M, Takemoto
K, Kato Y, et al. Absent cyclicity on aEEG within the first 24 h
is associated with brain damage in preterm infants. Neuropediatrics.
2010;41:241-5.
21. Hellström-Westas L, Rosén I, Svenningsen NW.
Predictive value of early continuous amplitude integrated EEG recordings
on outcome after severe birth asphyxia in full term infants. Arch Dis
Child. Fetal Neonatal Ed. 1995;72:F34-8.
22. Van Leuven K, Groenendaal F, Toet MC, Schobben
AF, Bos SA, de Vries LS, et al. Midazolam and
amplitude-integrated EEG in asphyxiated full-term neonates. Acta
Paediatr. 2004;93:1221-7.
23. Shany E. The influence of phenobarbital overdose
on aEEG recording. Eur J Paediatr. Neurol. 2004;8:323-5.
24. Tas JD, Mathur AM. Using amplitude-integrated EEG
in neonatal intensive care. J Perinatol. 2010;30(Suppl): S73-81.
25. Ter Horst HJ, Brouwer OF, Bos AF. Burst
suppression on amplitude-integrated electroencephalogram may be induced
by midazolam: A report on three cases. Acta Paediatr. 2004;93:559-63.
26. Foreman SW, Thorngate L, Burr RL, Thomas KA.
Electrode challenges in amplitude-integrated electro-encephalography (aEEG):
Research application of a novel noninvasive measure of brain function in
preterm infants. Biol Res Nurs. 2011;13:251-9.
|
|
 |
|