|
Indian Pediatr 2016;53: 889-899 |
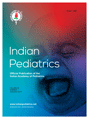 |
Point of Care Neonatal
Ultrasound — Head, Lung, Gut and Line Localization
|
Chandra Rath and *Pradeep Suryawanshi
From Departments of Neonatology, Royal North Shore
Hospital, Pacific High way, St Leonards, NSW, Australia; and *Bharati
Vidyapeeth University Medical college, Pune, Maharastra, India.
Correspondence to: Dr Pradeep Suryawanshi, Professor
and Head, Department of Neonatology, Bharati Vidyapeeth University
Medical College, Pune-Satara Road, Pune, Maharastra 411 043, India.
[email protected]
Received: July 25, 2015;
Accepted: June 11, 2016.
Published online: July 01, 2016. PII:S097475591600016
|
Context: Knowledge and skills of heart, head,
lung, gut and basic abdominal ultrasound is of immense utility to
clinicians in their day-to-day patient management, and in acute events,
in the absence of specialist service back-up. This review examines the
potential role of clinician-performed ultrasound in the neonatal
intensive care unit.
Evidence Acquisition: The bibliographic
search of English-language literature was performed electronically using
PubMed and EMBASE databases for the different topics we have covered
under this review.
Results: Bedside head ultrasound can be used
to identify and screen for intraventricular hemorrhage, periventricular
leukomalacia and post-hemorrhagic ventricular dilatation. It is also a
useful adjuvant tool in the evaluation of hypoxic ischemic
encephalopathy. The relatively new lung ultrasound technique is useful
in identifying transient tachypnea, pneumonia, pneumothorax, fluid
overload and pleural effusion. Gut ultrasound is useful in identifying
necrotizing enterocolitis and probably is better than X-ray in
prognostication. Ultrasound is also useful in identifying vascular line
positions without radiation exposure.
Main conclusions: Ultrasound performed by the
clinician has an extensive role in the neonatal intensive care unit.
Basic ultrasound knowledge of head, lung and gut is a useful supplement
to clinical decision-making.
Keywords: Decision-making, Evaluation, Neonatal
intensive care unit, Investigations.
|
Ultrasonography (USG) is no longer the exclusive
domain of radiologists and cardiologists. With appropriate training,
clinician performed ultrasound (CPU) is now practised widely in
obstetrics, emergency medicine and adult intensive care, and is the
standard practice in neonatology in many developed countries [1].
Cardiologists and radiologists undoubtedly have an indispensable role to
play in clinical care, but it is unrealistic to expect 24-hour
specialist cover, even in a resource-rich setting. Neonatal intensive
care is a dynamic process, involving frequent evaluation, some in real
time, which makes dependence on radiologist or cardiologist impractical.
CPU has already proven its mettle in day-to-day management and the
information obtained has often resulted in a management change [2]. In
this review, we shall discuss the practical use of ultrasound for
imaging the head, lung, and gut, and for vascular line localization. We
also discuss the application to clinical decision making in
resource-poor settings.
Cranial Ultrasonography
Role of cranial ultrasonography in neonatal intensive
care unit (NICU) is for:
• Preterm infants for evaluation of germinal
matrix hemorrhage-intraventricular hemorrhage (GMH-IVH) and
follow-up.
• Unexplained cardiac failure (to rule out
vascular abnormalities).
• Hypoxic ischemic encephalopathy (HIE).
• Congenital malformations.
• Neonatal seizures.
• Evaluation of suspected subgaleal hematoma
• Evaluation of antenatally detected
abnormalities.
Preterm infants, especially those less than 32 weeks
gestation, are at risk for GMH-IVH, and ischemic white matter injuries.
Late preterm infants who are monochorionic twins, small for gestational
age (SGA), and or have experienced events such as chorioamnionitis,
fetal distress, acidosis, difficult delivery, or hypotension are also at
risk for ischemic white matter injury. If these abnormalities are
detected early via ultrasound, follow-up and early intervention can be
planned appropriately. Serial ultrasounds may be necessary to detect
white matter lesions, which may not be evident until 2 to 4 weeks after
the ischemic event. There may be significant changes in USG findings
between the first and second scan, possibly changing medical management
and prognosis. Serial USG is also important in identifying significant
post-hemorrhagic hydrocephalus for early intervention.
Procedure
Cranial USG is done through the anterior and
posterior fontanelle, the mastoid foramen and poorly ossified parts of
the temporal bone. The mastoid, temporal and posterior fontanelle views
are supplementary to the absolutely necessary anterior fontenalle view.
Usually 5-10 Hz 2D curved or linear array transducers are useful for
cranial USG. Frequency of the probe may be increased for optimal
visualization of superficial structures like subcortical white matter
and venous sinuses; this will increase resolution at the expense of
penetration. Similarly, lower frequency may be used for visualization of
deeper structures in the posterior fossa. The transducers used should
fit perfectly on the anterior fontanelle, as a large footprint makes the
contact and image quality suboptimal, and small footprints reduce the
diagnostic ability.
In this review, we shall focus on hemorrhage,
parenchymal changes and hydrocephalous evaluation, which are most
frequently encountered in day-to-day practice.
Germinal Matrix-Intraventricular Hemorrhage
GMH-IVH is one of the most common ultrasound findings
in NICU. Studies performed in the 1980s suggested that >90% IVH cases in
very low birth weight (VLBW) infants occurred within postnatal days 4 to
5 [3]. Premature infants are relatively resistant to hemorrhage after
this period, irrespective of the gestational age (GA) because of the
shutdown in angiogenesis, making the vessels resistant to rupture
despite fluctuation in the cerebral blood flow [4]. A recently published
review [5] which included studies from the antenatal steroid and
surfactant era, concluded that 48% of cases of IVH occured in the first
6 hours of life in VLBW infants, and suggested that early cranial USG
may have prognostic, preventive and medicolegal implications. A small
percentage of GMH-IVH may occur up to third week of life.
Observational studies from the 1990s showed that in
the first two weeks of life, 12-51% of infants <1,500 grams or
gestational age of <33 weeks had abnormalities on ultrasound out of
which 6 -20% were major (such as grades 3 and 4 IVH or bilateral cystic
periventricular leukomalacia) [3]. More severe IVH occurs in more
premature infants. Although the American Academy of Neurology and the
Practice Committee of the Child Neurology Society [3] suggested
screening all preterms <30 weeks due to the incidence of severe IVH [3],
infants up to 34 weeks are also at increased risk of GMH-IVH. In a
recent study by Ballardini, et al. [6] in late preterm infants
(33-36 weeks), intracranial lesions were found in 13% of the neonates
when ultrasound was undertaken within day 7 of life. The risk factors
for detecting intracranial abnormalities were head circumference less
than the 3 rd percentile, the
need for ventilation or surfactant, low Apgar score at fifth minute, and
neurological abnormalities. However, severe grades of IVH and extreme
periventricular leukomalacia (PVL) are rare in this gestational age [6].
In another study, Bhat, et al. [7] detected abnormal cranial
ultrasonography in 6.8% preterm (30-34 weeks) newborns and recommended
screening in infants born between 30 and 34 weeks of gestational age.
They also detected severe intracranial anomalies in 1.5% of neonates in
this gestational age group; however, inclusion of less than 40% of the
eligible neonates [8], born during the study period makes this data a
little less meaningful. Vanderwalt, et al. [8] in a cost analysis
study, concluded that cranial ultrasound screening of infants
>32 weeks is not
cost-effective.
There is no consensus for the optimal timing for
cranial ultrasonography. Based on the above discussion, we propose a
screening schedule for preterm neonates (Table I). The
incidence of severe intracranial abnormalities is low in neonates with
gestational age greater than 30 weeks, and the proposed schedule may not
be very cost-effective in resource-poor settings. IVH is often
asymptomatic but the likelihood of signs increase with the severity of
hemorrhage. Possible clinical signs are: tense anterior fontanelle,
pallor and associated drop in hematocrit, unresponsiveness; tonic
seizures and decerebrate posturing; these should warrant immediate
bedside cranial USG.
TABLE I Proposed Cranial Ultrasonography Scanning Protocol for Preterm Infants
< 28 weeks or birth weight |
28-31+6 weeks or birth weight |
32-34 weeks with risk factors: Monochorionic
|
<1000g or 28-31+6 weeks |
1000-1500g without life support |
twins, head circumference <3rd centile, ventilation |
and/or birth weight <1500 g |
|
and/or surfactant need, fetal distress, acidosis, |
on life support. |
|
5 minute APGAR score of <6, or hypotension |
6 hours of age |
Day 3 to 1 week |
Day 5 to 1 week and then as indicated |
Day 3 to 1 week |
4 weeks |
|
4 weeks |
TAE or discharge |
|
Term age equivalent (TAE) |
|
|
or discharge whichever occurs first |
|
|
One week after any “new” sick event such as sepsis,
hypotension, necrotizing enterocolitis, etc. (If near term after
the 1 week scan then as required) [9]. In case of IVH other than
GMH alone, weekly scans are indicated. Cranial USG anytime in
case of clinical suspicion of IVH. |
Approximately 50-75% of preterm survivors with
predominantly grade IV IVH develop cerebral palsy, intellectual
disability, and/or hydrocephalus [10,11]. A recent Australian report on
neurodevelopmental outcomes of extremely preterm infants revealed that
grade I–II IVH, even in the absence of white matter injury or other late
ultrasound abnormalities, is associated with adverse neurodevelopmental
outcomes [12], supporting the use of routine cranial USG to identify all
silent GMH-IVH. A grading system developed by Papile and Burstein [13]
is still widely used for prognostication where grade I is a bleeding
confined to germinal matrix and looks as echogenic as choroid plexus in
USG. The caudothalamic groove acts as a convenient landmark:
echogenicity anterior to the groove represents blood as the choroid
finishes at the groove. Grade II is grade I with intraventricular
extension where blood can be seen as white bright spots/lines in the
ventricles separate from choroid plexus, grade III is ventricle
dilatation because of excessive blood inside it, and grade IV is
extension of hemorrhage in to the parenchyma. It must be remembered here
that Papile classification was originally developed using CT scan but
there have been reports of its use in cranial USG with accuracy [14].
Grade IV is interpreted as the result of an extension
of the hemorrhage from the ventricle into the adjacent white matter.
However, it is now postulated that large blood clots in the germinal
matrix and ventricles impair the flow of blood from the medullary veins
(which drain the cerebral white matter) into the terminal vein leading
to venous infarction and possibly hemorrhagic infarction i.e.,
periventricular hemorrhagic infarct (PVHI). Besides this compression
theory, ependymal trauma and inflammation as a possible cause has also
been proposed. Therefore, PVHI is not a simple extension of germinal
matrix hemorrhage into adjacent brain parenchyma as assumed in the
Papile classification [15]. PVHI is always associated with an
ipsilateral GM-IVH. When GM-IVH is bilateral, it usually is larger on
the side ipsilateral to the PVHI. A scoring system has been proposed
using parameters like the extent of PVHI, midline shift and unilateral
or bilateral PVHI. This scoring helps in better prognostication, as
there is a strikingly significant relationship between high PVHI score
and the likelihood to withdraw care, the development of early neonatal
seizures, and abnormal neuromotor examination at 12 and 30 months of age
[16,17]. Grade I-III IVH are easy to identify on cranial USG; however,
clinicians may occasionally face difficulties in differentiating PVL
from PVHI as both lesions are initially echogenic with later cystic
evolution. PVHI is an echodense lesion in the periventricular white
matter which is unilateral or, if bilateral, obviously asymmetric. PVHI
is also associated with a GMH-IVH lesion, which is usually ipsilateral
or larger on the ipsilateral side. PVL develops in the first week of
life as bilateral echo density at the lateral border of the lateral
ventricle with minimal or no IVH. PVHI usually evolves into a single or
few relatively large cysts, which communicate with the lateral ventricle
where as PVL evolves in to multiple tiny cysts, which do not communicate
with lateral ventricle. Bass, et al. [18] could differentiate
between PVHI and PVL in 77% of their study subjects with cranial USG
while 11% had mixed lesions [18].
Once the diagnosis of GMH-IVH is made, we should look
for cerebellar bleeding, as the external layer of the cerebellum is also
a germinal zone. Bleeding in and around the cerebellum may lead to poor
future neurodevelopmental outcome. Early detection with the help of
cranial USG through the mastoid foramen is important for prognostication
and appropriate counselling of the family. Though small punctate
cerebellar hemorrhages may not be seen well with cranial USG as compared
to MRI, it remains a useful bedside tool [19].
Post-hemorrhagic Ventricular Dilatation
The risk of developing post-hemorrhagic ventricular
dilatation (PHVD) is considerable after a severe hemorrhage (grade
III/IV). PHVD is defined as ventricular enlargement
³97th centile for
gestational age (GA) [20], and is recognized in about one-third of
infants with GMH-IVH. About 35% of neonates who develop PHVD require
some form of intervention [21]. Evaluation of progressive PVHD with
clinical parameters such as serial measurement of head circumference,
tense fontanelle, sunset phenomena of the eyes are not as reliable as
serial cranial USG [20,22,23]. It is hard to distinguish
post-hemorrhagic ventri-culomegaly from atrophic ventriculomegaly
resulting from white matter loss. However, regardless of the mechanism,
the extent of white matter loss has a direct correlation with the motor
outcome [24]. The measurements commonly used in clinical practice (Fig.
1) are accurate compared to MRI [25]. Ventricular index is one of
the most commonly used measurements and the reference value correlates
well for term neonates. However, it may not increase during early
hydrocephalus and the reference values for preterm infants show
variation because of less representation of this population in the
reference curves [20,26-28]. Another commonly used measurement, the
anterior horn width (AHW), has the advantage of identifying early
hydrocephalous [27] with a minimal variation with change in gestational
age [27,29]. However, a recent study by Sondhi, et al. [30]
demonstrated an evident increase in size with ongoing maturity. Thalamo-occipital
distance (TOD), which essentially measures the occipital horn length of
the lateral ventricle, may be a useful measurement and sometimes
represent the only site of ventricular dilatation [30]. Absence of
increased TOD is an important negative finding. However, difficult
visualization, considerable variation in reference curves, and the
presence of isolated dilation of the occipital horn in normal preterm
infants makes this measurement clinically less meaningful [29-31]. Other
measurements like ventricular height and frontal horn ratio are less
valuable in clinical practice as no reference curves are available.
Measurement of the 3rd and 4th
ventricle may assist in differentiating communicating and
non-communicating hydrocephalus; however, the absence of quality
reference curves, inter-observer variability, and difficulties in
measurements are the main drawbacks [29,30].
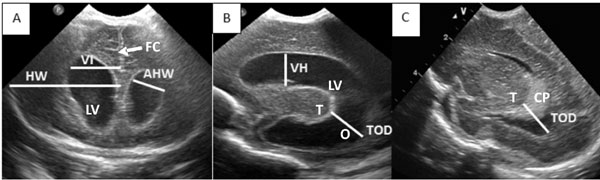 |
Fig. 1 Commonly used
measurements used in evaluation of PHVD.A-Coronal plane
Ventricular Index (VI)- Distance between the falx and the
lateral wall of the anterior horn at the level of the third
ventricle (4 mm above the 97th centile for GA is an indication
for CSF drainage), FHR = VI/Hemispheric width (HW), AHW-Maximum
diagonal width (values above 6mm significant). B- Sagittal plane
TOD- Distance between the outermost point of the thalamus at its
junction with the choroid plexus, to the outermost part of the
occipital horn, Ventricular height (VH)- At the level of foramen
of Monro. C- Sagittal plane- TOD in a non-dilated ventricle, LV-
Lateral ventricle, T- Thalamus, CP- Choroid plexus, O- Occipital
horn of the lateral ventricle, FC- Falx cerebri
|
Ventricular index and AHW are the most widely studied
and used measurements in clinical practice. Vetricular index greater by
4 mm of the 97th centile for gestational age is associated with a poor
prognosis [21]. A normal AHW is less than 3 mm, with the 95th percentile
curve reaching 2 mm at 36 weeks and 3 mm at 40 weeks. A size of more
than 6 mm is considered abnormal. The implications of AHW between 3 and
5 mm is not clear. Cranial USG is useful in the identification of PVHD
and should be undertaken at least twice weekly to identify progression;
however interventional decisions are usually a combination of clinical
findings, history and ultrasound findings. Indian data regarding these
measurements are scarce [32,33].
Periventricular Leukomalacia
PVL, which occurs as a consequence of preterm brain
ischemia and/or inflammation, is of great diagnostic importance because
of its association with cerebral palsy and abnormal development. PVL
usually occurs in preterm infants £32
weeks gestation as they have poorly vascularized white matter, which
contains oligodendro-cyte progenitors sensitive to ischemia and
inflammation [34]. MRI has been reported to be a better modality than
ultrasound in detecting white matter injury particularly in the
diagnosis of punctate white matter lesion (PWML) and diffuse excessive
high signal intensity [35]. However, serial USG has a definite role in
evaluation of cystic PVL, a more severe form of white matter injury. The
more extensive cysts tend to develop within 2-3 weeks following an
insult, while the more localized cystic lesions may take as long as 3-6
weeks to develop [36]. Therefore, PVL diagnosed in the first week of
life indicates an antenatal insult rather than a perinatal insult.
Echogenicity in the brain equal to or greater than echogenicity in the
choroid plexus, when persisting for more than 10-14 days, should alert
the clinician about possible early PVL. Transient hyper-echoic lesions
or periventricular halos might be seen in normal white matter of preterm
infants. The pattern of distribution of PVL on ultrasound is typically
dorsal and lateral to the external angles of the lateral ventricles. Any
brain lesion, which causes brain parenchymal loss, may result in cyst
formation. It has been suggested that PVHI and PVL can be differentiated
by the location of the cysts. PVL has a predilection for periventricular
arterial border zones, particularly in the region near the trigon of the
lateral ventricles. PVHI is prominent more anteriorly with the lesion
radiating from the periventricular region at the site of confluence of
the medullary and terminal vein and assumes a triangular, fan-shaped
appearance in the periventricular white matter [14]. The typical
positions of various cystic lesions are depicted in Fig. 2.
A classification for PVL has been suggested, though this is not widely
accepted [37].
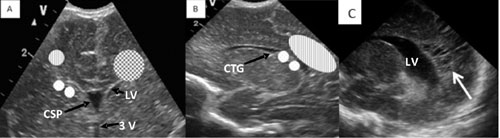 |
Fig. 2 A- Coronal view- Dotted
area- Site for subependymal cyst, connatal cyst, Striped – Site
for PVL, Chequerboard- Site for PVHI. B- Sagittal view- Striped-
Site for PVL, Dotted area- site for choroid plexus cyst. C-White
arrow showing cystic PVL. LV- Lateral ventricle, 3V-3 rd
Vetricle, CSP- Cavum septum pellucidum, CTG-Caudothalamic
groove.
|
Doppler Evaluation
Doppler imaging of the anterior cerebral artery (ACA)
and middle cerebral (MCA) is easily done through the anterior fontanelle
in the sagittal plane and through the temporal window in the axial
plane. The peak systolic velocity (PSV), end diastolic velocity (EDV),
resistive index (RI) and pulsitility index (PI) are the most common
measurements used for monitoring intracranial haemodynamics. Measuring
PI is useful as it minimizes the effect of vessel angulation and
correlates well with acute changes in intra-cerebral perfusion pressure
[38]. Age-dependent reference values are available, and the normal range
for the RI is 0.65 - 0.90. Values <0.5 or >0.9 are abnormal. An increase
in diastolic flow results in a decrease in the RI, and conversely a
decrease in diastolic flow results in an increase in the RI. Various
factors can influence RI; for example, presence of a patent ductus
arteriosus (PDA), scanning pressure on the anterior fontanelle, IVH,
PVL, hydrocephalus, pneumothorax and low arterial carbon dioxide can
increase the RI. Similarly RI is decreased in asphyxia, vascular
malformation, tachycardia and decreased cardiac output [39]. RI <0.5 in
asphyxiated newborns in the first few days of life is associated with
both immediate and long term poor outcome [40-45]. Unfortunately, some
full-term neonates with significant asphyxia may not show this decreased
RI and may instead have a normal or increased RI which may be due to a
relative decrease in diastolic flow velocity. This decrease in diastolic
flow velocity may be because of the presence of a significant PDA,
myocardial dysfunction (such as in transient myocardial ischemia), or
hypervolemia. Mean cerebral blood flow is mainly determined from the
diastolic flow. As intracranial pressure (ICP) rises, the arterial flow
is more affected during diastole than during systole, resulting in an
increase in RI as happens in hydrocephalus [46]. In individual infants,
a tendency towards a correlation between ICP and flow variables was
found when studied longitudinally [47]. However, it is doubtful whether
the RI can be used as an indicator for the timing of intervention,
because it can vary widely between individual preterm infants and
accuracy subjected to presence of other conditions, that may influence
cerebral blood flow.
Cranial USG has many other applications in term and
preterm infants which is beyond the scope of this review. It is
excellent for the detection of IVH, ventriculomegaly, perforator stroke,
sinovenous thrombosis and cystic PVL, but MRI is superior in detecting
cortical abnormalities, posterior fossa lesions, subtler white matter
injury, early watershed infarct events, microabscesses and involvement
of posterior limb of internal capsule. Reviews of the studies directly
comparing cranial USG with MRI with cerebral palsy as the outcome show
that utility of MRI tends to be similar or higher compared with cranial
USG [48,49]. In a recently published study, serial cranial USG seems
highly effective in diagnosing all common preterm brain injuries, but
may miss cerebellar abnormalities [50]. However, it will be interesting
to see neurodevelopmental prediction with early MRI in few of the
upcoming studies.
Lung Ultrasound
Clinical signs and radiographs are routinely used to
diagnose neonatal lung disease albeit they have low specificity and
sensitivity for many common clinical conditions. Lung ultrasound is
being increasingly used in Neonatal Intensive Care Unit (NICU) and adult
ICU because of its high sensitivity and specificity [51]. It is easy to
learn and can be performed with a basic ultrasound machine. Lung and
pleura being superficial structures, USG requires a high-frequency
linear array probe (>7.5 MHz). Micro convex probe may be used, however,
linear probe displays a wider field. A basic USG setting with 2D, M mode
and occasional color Doppler is all that is required to do lung
ultrasound. Normal USG of lung shows ‘A lines’, which are parallel to
straight solid pleural lines, are reverberation artefacts, and are
equidistant from each other. On the other hand B-lines occur when sound
waves pass through the pleural line encountering a mixture of air and
water as in pulmonary oedema. These are discrete laser-like vertical
hyper echoic lines that arise from the pleural line, extend to the
bottom of the screen without fading, and move synchronously with lung
sliding (Fig. 3). The pleural line slides from side to
side with respiration and represents movement of the pleural surface
with the respiratory cycle. This sign is known as sliding sign, a normal
lung feature. Lung sliding can also be observed using time motion mode
(M mode) where the fixed superficial chest wall structures give rise to
an appearance of water and the constantly moving underlying lung gives
rise to a sandy appearance known as seashore sign (Fig. 3).
The ‘lung pulse’ refers to the rhythmic movement of the pleura in
synchrony with the cardiac rhythm. As the heart beats the movement of
the heart is transmitted through the medium of the lung, which is
demonstrated in M-mode as a regular motion artefact through the seashore
pattern to the level of the pleura. In normal well-aerated lung, the
‘lung pulse’ is not present, as lung sliding becomes dominant and
resistant to cardiac vibrations. The lung pulse is easily identified
when the baby is not breathing.
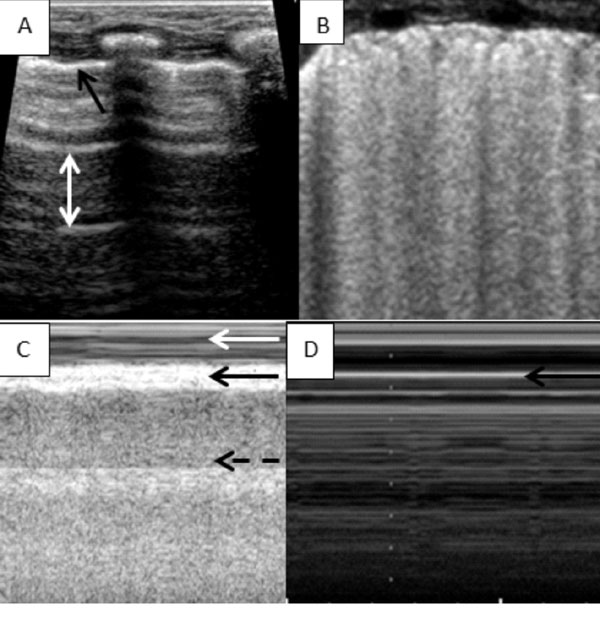 |
Fig. 3 (A) Normal lung ultrasound-
Horizontal A lines shown by the white arrow equidistance from
each other and the pleural line, black arrow showing the pleural
line (PL). (B) Multiple vertical lines starting from the PL and
almost coalescing with each other giving a white lung
appearance. (C) Normal lung M mode- Seashore sign, black arrow
denoting PL, white arrow showing the chest wall looking like
water and the broken black arrow showing lung parenchyma looking
like a sandy beach. (D) Stratosphere sign as seen in
pneumothorax, there is no water and sandy part, it all looks
like water.
|
Pneumothorax
USG is an invaluable tool for the assessment of
pneumothorax, with accuracy approaching CT, and far exceeding plain
radiography in adults [52]. It is of immense value in emergencies such
as tension pneumothorax, as it is readily available at the bedside and
can be done in less than a minute. Features of pneumothorax such as the
absence of lung sliding, presence of lung point (A point where seashore
sign changes in to stratosphere sign), presence of stratosphere sign on
M mode (Fig. 3), absence of B-lines and absence of lung
pulse are easy to identify with minimal training. In Stratosphere sign
parallel horizontal lines above and below the pleural line is noted and
it resembles a barcode. In contrast to the seashore sign which is a
normal lung sign, in stratosphere sign the grainy shore below the
pleural line is not seen (which is due to the movement of the lungs with
respiration), rather only sea (parallel lines) is noted and this denotes
a static lung which is not moving with respiration because of
pneumothorax (Fig. 3). Though studies in neonates are
lacking, Lichtenstein, et al. [53] from his experience in NICU
suggested that neonatal signs are no different from adult lung signs.
Pneumonia
Lung ultrasound is a clinically useful tool in
diagnosing pneumonia; however, consolidation that does not reach the
pleura cannot be visualised. In adults, lung consolidation extends to
the pleura in 98.5% of cases and can be seen on USG [52]. Lung mass is
smaller in the newborn and extension to the pleura may be much more
frequent. Coarse and/or irregular disrupted pleural line, hepatisation
of the lung tissue (echogenicity similar to liver), hyperechoic area of
varying size and shape in the same lung field, irregular margin around
consolidation, presence of dynamic air-bronchogram, disappearance of
lung sliding, mild pleural effusion and presence of lung pulse are few
of the features which can be identified in pneumonia. The international
consensus committee on lung ultrasound agreed that there is strong
evidence that USG is an accurate tool in diagnosing lung consolidation
when compared with chest radiography in pediatric age group [54]. Some
neonatal studies have also shown USG to be a useful tool in recognizing
neonatal pneumonia with good specificity and sensitivity [55,56].
Pleural Effusion
Opacities detected by conventional radiography can be
differentiated as consolidation or effusion only by an ultrasound scan.
For pleural effusions, USG has a sensitivity of 93% and specificity of
97% [57]. USG can also be used to differentiate between transudate and
exudate [54]. Visualization of internal echoes, mobile particles or
septa, is highly suggestive of exudate; however, in case of an anechoic
effusion, the only way to differentiate between transudate and exudate
is to use thoracoentesis.
Extravascular Fluid
Presence of vertical ‘B lines’ represents
extravascular fluid in lungs. (Fig. 3). B lines can be
used to monitor cardiac failure (systolic and diastolic), iatrogenic
fluid overload (a sudden change from A to B line), or preload/afterload
reduction therapy. However, B lines and white lung in neonates should be
considered in the clinical context of the disease. B lines and white
lung can also be seen in respiratory distress syndrome (RDS), transient
tachypnea of the newborn (TTN), consolidation and atelectasis of any
cause, meconium aspiration syndrome and broncho-pulmonary dysplasia.
Respiratory Distress Syndrome and Transient Tachypnea
of the Newborn
USG is a useful tool in the management of RDS and TTN
with good interobserver agreement. In TTN, very compact B lines in the
inferior pulmonary fields and not so compact B lines in the superior
lung field gives a characteristic sign called the double lung point, a
sign with which we may use to differentiate it from RDS. The double lung
point sign is also useful in management and prognosis, particularly in a
resource-poor setting. Co-existence of lung consolidation, abnormal
pleural line (thickness of >0.5mm or blurred), bilateral white lung and
disappearance of A lines are constant ultra-sonography features of RDS
with a specificity and sensitivity of 100%. Other features like pleural
effusion, lung pulse and uniform bilateral involvement are infrequent
associations. The most important indicator of RDS is consolidation,
which is seen in all RDS patients but the extent and scope of
consolidation varies with severity of RDS. Consolidation in moderate RDS
is sub pleural and focal in nature whereas consolidation in severe RDS
is more widespread and deep.Similarly lung pulse was present in all
grade 3 and 4 RDS while it was absent in all grade 2 RDS [58]. In term
and near term infants, USG at 1-2 hours of life has been shown to
anticipate the need for respiratory supportand severe respiratory
distress with 100% specificity and 77.7% sensitivity [59]. In a recent
study, lung ultrasound predicted need for intubation after 2 hours of
life in preterm babies with a positive predictive value of 100%, and
negative predictive value of 94.7% [60]. Another recent study predicted
the need for Surfactant administration on the basis of a scoring system
which consists of oxygenation indices and lung ultrasound, with a
sensitivity and specificity of 100% and 61% respectively [61]. The basic
principle in all these studies is the abundance of B lines. A higher
number of B lines appear as whiter lungs that need more support compared
to a finding with more A lines.
USG is not yet completely ready to replace X-ray
in neonatology. Few non-specific signs, paucity of neonatal research and
publications are the drawbacks. However, this technology undoubtedly has
the potential to replace X-ray as the most useful bedside lung
disease diagnostic tool.
Necrotizing Enterocolitis (NEC)
Identification and management of NEC is currently
based on recommendations from the modified Bell’s criteria [62].
Abdominal X-ray is the cornerstone in diagnosis and is able to
detect bowel distension, bowel wall thickness, pneumatosis intestinalis,
portal venous gas and free abdominal air. USG provides additional
information about gut viability and free fluid in the abdomen. An 8-15
MHz linear probe should be used for bowel loop ultrasound.
Data on normal thickness of the bowel in preterm
neonates is scarce; we suggest a thickness of 1.2 to 2 mm from personal
experience. Normal term bowel wall thickness has been described as 1.1
to 2.6 mm. A normal bowel perfusion is 1–9 colour doppler signal dots
per cm2 (mean 3.8) in a
setting of the lowest possible pulse repetition frequency and the
highest Doppler gain settings without flash artefacts. The velocity was
set at 0.029 – 0.11 m/sec [63]. Normal bowel wall is smooth with
peristalsis.
A bowel wall thickness >2 mm should be considered
suspicious and conversely; a thickness <1.0 mm indicates an abnormal
thinning resulting from ischemia or necrosis. Increased bowel perfusion
may present in different patterns such as ring-shape, Y-shaped and
zebra-shaped. Absent bowel perfusion can be assumed when no color signal
is detected at the slowest possible velocity (0.029 m/sec) and suggests
a complete bowel wall necrosis with 100% sensitivity [63]. Intramural
gas, a common finding though not pathognomonic of NEC, can be identified
as highly echogenic dots in the bowel wall and may involve the whole
circumference, in which case it is called the "circle sign" (Fig. 4).
Intramural gas must be differentiated from intraluminal gas, which moves
with compression of the abdomen with the ultrasound probe. The amount of
intramural gas present does not always relate to the clinical severity
of NEC and its disappearance does not correlate with clinical
improvement [64]. In the absence of NEC, the commonest cause of portal
venous gas is the passage small amounts of gas through an umbilical
venous catheter. Neither is the presence portal venous gas fatal, nor
does its disappearance always herald clinical improvement. Portal venous
gas has been reported in only 30% of the neonates with NEC, and is
detected by ultrasound much earlier than it appears on X-ray
[65,66]. Free abdominal gas secondary to perforation can been seen as a
bright white hyper echogenicity between the diaphragm and liver which
moves with abdominal compression. Detection of intra peritoneal fluid
and or a mass may help in diagnosing perforated NEC. In a study by
Silva, et al. [67], when three of the seven USG features (portal
venous gas, intramural gas, increased wall echogenicity, bowel wall
thickening or thinning, absent perfusion, free echogenic fluid) were
present, there was a sensitivity of 0.82 and a specificity of 0.78 for
poor outcome.
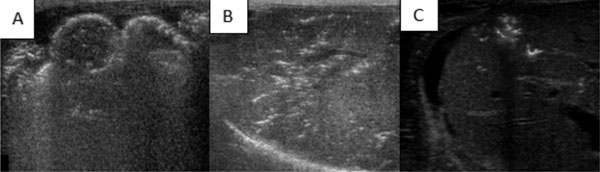 |
Fig. 4 (A) Extensive pneumatosis intestinalis (white
dots) white arrow- Bowel wall, (B) Extensive portal venous air
in the liver USG (White dots), (C) Portal venous gas (White
dots).
|
USG for NEC is not without drawbacks; inter-observer
variability, large amount of bowel gas and tender unstable abdomen may
hamper good USG evaluation. However, USG has an obvious advantage over
routine X-ray in diagnosis and prognostication of NEC [68],
especially in neonates with clinical deterioration without X-ray
changes.
Line-Localization
Though central line placement in NICU is a necessity,
it is not without complication. Identification of central line tip
location may help in reducing the complications, and USG is one of the
easiest bedside modality to do so. A recently published review [69]
suggested considering USG as a potential alternative to X-ray in
central line tip location in neonates. Two recent studies [70,71] could
identify around 25% of the cases with abnormal tip position, which were
reported to be normal in X-ray reporting. Ultrasound-guided
umbilical catheter placement is a faster method to place catheters
requiring fewer manipulations and X-rays when compared with
conventional catheter placement [72].
Training and Medico-legal Implications
It is important to have a structured training program
for clinicians in order to make them ultrasound literate. Few developed
countries in the world have a structured training program for bedside
echocardiography and fewer have it for bedside cranial ultrasound
[1,73]. Most of these training programs require the clinician to
undertake 75-250 studies under the guidance of the experts in an
accredited center, and which might take anytime between 6 months to 24
months to complete. The course also includes hands on basic, advanced
training courses and an online physics course.
However, issues like different clinical needs,
misdiagnosis, medicolegal liability and financial return for
examination, need further discussion. Clinical need is a pertinent issue
in the Indian scenario, as there are very few hospitals around the
country catering to newborns that have in-house radiological and
pediatric cardiology services. In reality, 24-hour presence of
specialists to provide ultrasound services in the NICU is not
achievable. It is here where clinician-performed ultrasound can be
handy. However, the risk of misdiagnosis is a real and important
concern, and some of this can be resolved by guidelines about when
consultative referral should be mandatory. The other important step,
which can reduce misdiagnosis, is structured training and accreditation.
There is a medico-legal vacuum as far as clinician- performed ultrasound
is concerned. If a registered medical practitioner with six months
training or one year experience in sonography or a gynecologist with
experience are allowed to do ultrasound, we do not see any reason why
adequately trained clinicians cannot do bedside USG for better patient
management. The motivation to acquire point of care ultrasound skill
should be to assist in clinical decision-making and clinicians should be
careful in practicing outside the limits of their skills. Neonatologists
with adequate training should be able to report his/her USG findings in
the progress sheet for day-to-day clinical decision-making. It is
important to mention in the report, whether clinician or an imaging
specialist performs bedside ultrasound.
Conclusion
Head, lung and abdomen ultrasound are useful bedside
clinical tools, which can be used as frequently as required without the
risk of radiation exposure. Cranial USG is most commonly used to
identify IVH, PVHD and cystic PVL with good efficacy. Lung ultrasound is
useful in identifying pneumothorax, pleural effusion, pneumonia and
plays a supportive role in the management of RDS. Bedside USG for NEC
should be supplementary to usual management. Bedside USG has a definite
role in line localization. Use of bedside USG in neonatology is on the
rise with frequent new utility additions like endotracheal tube tip
localization and is becoming an obligatory screening and diagnostic
tool.
It must be emphasized here that clinician-performed
USG is not here to replace the role of pediatric cardiologists and
radiologists in neonatal practice. However, ultrasound-literate
clinicians should be able to do USG in an acute clinical setting,
document it and do appropriate intervention in absence of specialist
expertise.
Contributors: Both authors contributed to
literature search, manuscript writing and its approval.
Funding: None; Competing interest: None
stated.
References
1. Evans N, Gournay V, Cabanas F, Kluckow M, Leone T,
Groves A, et al. Point-of-care ultrasound in the neonatal
intensive care unit: international perspectives. 2011;16:61-8.
2. El-Khuffash A, Herbozo C, Jain A, Lapointe A,
McNamara PJ. Targeted neonatal echocardiography (TnECHO) service in a
Canadian neonatal intensive care unit: a 4-year experience. J Perinatol.
2013;33:687-90.
3. Ment LR, Bada HS, Barnes P, Grant PE, Hirtz D,
Papile LA, et al. Practice parameter: neuroimaging of the
neonate: report of the Quality Standards Subcommittee of the American
Academy of Neurology and the Practice Commit- tee of the Child Neurology
Society. Neurology. 2002;58:1726-38.
4. Praveen B. Pathogenesis and prevention of
intraventricular hemorrhage. Clin Perinatol. 2014;41:47-67.
5. Al-Abdi SY, Al-Aamri MA. A systematic review and
meta-analysis of the timing of early intraventricular hemorrhage in
preterm neonates: Clinical and research implications. J Clin Neonatol.
2014;3:76-88.
6. Ballardini E, Tarocco A, Baldan A,( Antoniazzi E,
Garani G, Borgna-Pignatti C. Universal cranial ultrasound screening in
preterm infants with gestational age 33-36 weeks. A retrospective
analysis of 724 newborns. Pediatr Neurol. 2014;51:790-4.
7. Bhat V, Karam M, Saslow J, Taylor H, Pyon K,
Kemble N, et al. Utility of performing routine head ultrasound in
preterm infants with gestational age 30-34 weeks. J Matern Fetal
Neonatal Med. 2012;25:116-9.
8. Van der walt C, Vazzalwar R, Schweig L, Donovan R.
IVH Screening By Cranial Ultrasound for All Preterm Infants
³30 Weeks Is Not Cost
Effective. In: Proceedings of the AAP Experience National
Conference & Exhibition: Perinatal Pediatrics Scientific Posters
Presentations; 2013 October 25; Orlando. Florida; 2013. Available from:
https://aap.confex.com/aap/2013/webprogram/Paper 21331.html.
Accessed July 15, 2015.
9. Andre P, Thebaud B, Delavaucoupet J, Zupan V,
Blanc N, d’Allest AM, et al. Late-onset cystic periventricular
leukomalacia in premature infants: a threat until term. Am J Perinatol.
2001;18:79-86.
10. Sherlock RL, Anderson PJ, Doyle LW. Neuro-developmental
sequelae of intraventricular haemorrhage at 8 years of age in a regional
cohort of ELBW/ very preterm infants. Early Hum Dev. 2005;81:909-16.
11. Luu TM, Ment LR, Schneider KC, Katz KH, Alan WC,
Vohr BR. Lasting effects of preterm birth and neonatal brain hemorrhage
at 12 years of age. Pediatrics 2009;123: 1037-44.
12. Bolisetty S, Dhawan A, Abdel-Latif M, Bajuk B,
Stack J, Lui K. Intraventricular hemorrhage and neuro-developmental
outcomes in extreme preterm infants. Pediatrics. 2014;133:55-62.
13. Burstein J, Papile LA, Burstein R.
Intraventricular hemorrhage and hydrocephalus in premature newborns: A
prospective study with CT. Am J Roentgenol. 1979;132:631-5.
14. Khan IA, Wahab S, Khan RA, Ullah E, Ali M.
Neonatal intracranial ischemia and hemorrhage: Role of cranial
sonography and CT scanning. J Korean Neurosurg Soc. 2010;47:89-94.
15. Volpe JJ. Intracranial Hemorrhage. In:
Volpe JJ. Neurology of the Newborn. 5th Ed. Saunders; Philadelphia.
2008;11:517-88.
16. Bassan H, Benson CB, Limperopoulos C, Feldman HA,
Ringer SA, Veracruz E, et al. Ultrasonographic features and
severity scoring of periventricular hemorrhagic infarction in relation
to risk factors and outcome. Pediatrics. 2006;117:2111-8.
17. Bassan H, Limperopoulos C, Visconti K, Mayer DA,
Feldman HA, Avery L, et al. Neurodevelopmental outcome in
survivors of periventricular hemorrhagic infarction. Pediatrics.
2007;120:785-92.
18. Bass WT, Jones MA, White LE, Montgomery TR,
Karlowicz MG. Ultrasonographic differential diagnosis and
neurodevelopemental outcome of cerebral white matter lesions in
premature infants. J Perinatol. 199;19:330-6.
19. Steggerda SJ, Leijser LM, Wiggers-de Bruïne FT,
van der Grond J, Walther FJ, van Wezel-Meijler G. Cerebellar injury in
preterm infants: incidence and findings on US and MR images. Radiology.
2009;252:190-9.
20. Levene MI. Measurement of the growth of the
lateral ventricles in preterm infants with real-time ultrasound. Arch
Dis Child. 1981;56:900-4.
21. De Vries LS, Liem KD, van Dijk K, Smit BJ, Sie L,
Rademaker KJ, et al. Early versus late treatment of
posthaemorrhagic ventricular dilatation: results of a retrospective
study from five neonatal intensive care units in The Netherlands. Acta
Paediatr. 2002;91:212-7.
22. Ingram MC, Huguenard AL, Miller BA, Chern JJ.
Poor correlation between head circumference and cranial ultrasound
findings in premature infants with intraventricular hemorrhage. J
Neurosurg Pediatr. 2014;14:184-9.
23. Muller WD, Urlesberger B. Correlation of
ventricular size and head circumference after severe intra-periventricular
haemorrhage in preterm infants. Childs Nerv Syst. 1992;8:33-5.
24. Brouwer A, Groenendaal F, van Haastert I,
Rademaker K, Hanlo P, de Vries LS. Neurodevelopmental outcome of preterm
infants with severe intraventricular hemorrhage and ther- apy for
post-hemorrhagic ventricular dilatation. J Pediatr 2008;152:648–54.
25. Leijser LM, Srinivasan L, Rutherford MA, Counsell
SJ, Allsop JM, Cowan FM. Structural linear measurements in the newborn
brain: accuracy of cranial ultrasound compared to MRI. Pediatr Radiol.
2007;37:640-8.
26. Grasby DC, Esterman A, Marshall P. Ultrasound
grading of cerebral ventricular dilatation in preterm neonates. J
Paediatr Child Health. 2003;39:86-90.
27. Liao MF, Chaou WT, Tsao LY, Nishida H, Sakanoue
M. Ultrasound measurement of the ventricular size in newborn infants.
Brain Dev. 1986;8:262-8.
28. Brouwer MJ, de Vries LS, Groenendaal F, Koopman
C, Pistorius LR, Mulder EJH, et al. New reference values for the
neonatal cerebral ventricles. Radiology. 2012;262: 224-33.
29. Davies MW, Swaminathan M, Chuang SL, Betheras FR.
Reference ranges for the linear dimensions of the intracranial
ventricles in preterm neonates. Arch Dis Child Fetal Neonatal Ed.
2000;82:F218-23.
30. Sondhi V, Gupta G, Gupta PK, Patnaik SK, Tshering
K. Establishment of nomograms and reference ranges for intracranial
ventricular dimensions and ventriculo-hemispheric ratio in newborns by
ultrasonography. Acta Paediatr. 2008;97:738-44.
31. Reeder JD, Kaude JV, Setzer ES.The occipital horn
of the lateral ventricles in premature infants.An ultrasonographic
study. Eur J Radiol. 1983;3:148-50.
32. Chowdhary V, Culati P, Arora S, Thirupuram S.
Cranial sonography in preterm infants. Indian Pediatr. 1992;27:411-5.
33. Soni JP, Gupta BD, Soni M, Singh RN, Purohit NN,
Gupta M, et al. Normal parameters of ventricular system in
healthy infants. Indian Pediatr. 1995;32:549-55.
34. Blumenthal I. Periventricular leucomalacia: A
review. Eur J Pediatr. 2004;163:435-42.
35. Hart AR ,Whitby EW, Griffiths PD, Smith MF.
Magnetic resonance imaging and developmental outcome following preterm
birth: review of current evidence. Dev Med and Child Neurol.
2008;50:655-63.
36. De Vries LS, van Haastert IL, Rademaker KJ,
Koopman C, Groe- nendaal F. Ultrasound abnormalities preceding cerebral
palsy in high-risk preterm infants. J Pediatr. 2004;144:815-20.
37. De Vries LS, Eken P, Dubowitz LM. The spectrum of
leukomalacia using cranial ultrasound. Behav Brain Res. 1992;49:1-6.
38. Seibert JJ, McCowan TC, Chadduck WM, Adametz JR,
Glasier CM, Williamson SL, et al. Duplex pulsed doppler US versus
intracranial pressure in the neonate. Clinical and experimental studies.
Radiology. 1989;171:155-60.
39. Bulas DI. Transcranial doppler: Applications in
neonates and children. Ultrasound Clin. 2009;4:533-51.
40. Liu J, Cao HY, Huang XH, Wang Q. The pattern and
early diagnostic value of Doppler ultrasound for neonatal
hypoxic-ischemic encephalopathy. J Trop Pediatr. 2007;53:351-4.
41. Nishimaki S, Iwasaki S, Minamisawa S, Seki K,
Yokota S. Blood flow velocities in the anterior cerebral artery and
basilar artery in asphyxiated infants. J Ultrasound Med. 2008;27:955-60.
42. Argollo N, Lessa I, Ribeiro S. Cranial Doppler
resistance index measurement in preterm newborns with cerebral white
matter lesion. J Pediatr (Rio J). 2006;82:221-6.
43. Ilves P, Lintrop M, Metsvaht T, Vaher U, Talvik
T. Cerebral blood-flow velocities in predicting outcome of asphyxiated
newborn infants. Acta Paediatr 2004;93: 523-8.
44. Kirimi E, Tuncer O, Atas B, Sakarya ME, Ceylan A.
Clinical value of color doppler ultrasonography measure-ments of
full-term newborns with perinatal asphyxia and hypoxic ischemic
encephalopathy in the first 12 hours of life and long-term prognosis.
Tohoku J Exp Med. 2002;197:27-33.
45. Ilves P, Talvik R, Talvik T. Changes in doppler
ultrasonography in asphyxiated term infants with hypoxic-ischaemic
encephalopathy. Acta Paediatr. 1998;87:680-4.
46. Mackamee LR, Gonzales JI, Chance GW. Cerebral
blood flow velocity profiles in intraventricular haemorrhage progressing
to hydrocephalus. Pediatr Res. 1998;43:224A.
47. Maertzdorf WJ, Vles JSH, Beuls E, Mulder ALM,
Blanco CE. Intracranial pressure and cerebral blood flow velocity in
preterm infants with post-haemorrhagic ventricular dilatation. Arch Dis
Child Fetal Neonatal Ed. 2002;87:3 F185-8.
48. Soo HK, Lana V, Laura RM, Petra SH. The role of
neuroimaging in predicting neurodevelopmental outcomes of preterm
neonates. Clin Perinatol. 2014;41:257-83.
49. deVries LS, Benders MJ, Groenendaal F. Imaging
the premature brain: ultra- sound or MRI? Neuroradiology. 2013;55:13-22.
50. Plaisier A,( Raets MMA, Ecury-( Goossen GM,
Govaert P, Feijen-Roon M, Reiss IK, et al. Serial cranial
ultrasonography or early MRI for detecting preterm brain injury? Arch
Dis Child Fetal Neonatal Ed. 2015;100:F293-F300.
51. Lichtenstein DA, Mauriat P. Lung ultrasound in
the critically Ill neonate. Curr Pediatr Rev. 2012:8:217-23.
52. Lichtenstein D. Lung ultrasound in the critically
ill. Clin Intensive Care. 2005;16:79-87.
53. Lichtenstein DA. Ultrasound examination of the
lungs in the intensive care unit. Pediatr Crit Care Med. 2009;10:693-8.
54. Volpicelli G, Elbarbary M, Blaivas M,
Lichtenstein DA, Mathis G, Kirpatrick AW, et al. International
Liaison Committee on Lung Ultrasound (ILC-LUS) for International
Consensus Conference on Lung Ultrasound (ICC- LUS). International
evidence-based recommendations for point of-care lung ultrasound.
Intensive Care Med. 2012;38:577-91.
55. Hadeel M. Seif El Dien , Dalia AK ElLatif A. The
value of bedside Lung Ultrasonography in diagnosis of neonatal
pneumonia. Egyptian J Radiol Nuclear Med. 2013;44: 339-47.
56. Liu J, Liu F, Liu Y, Wang HW, Feng ZC. Lung
Ultrasonography for the diagnosis of severe neonatal pneumonia. Chest.
2014;146:383-8.
57. Lichtenstein D, Goldstein I, Mourgeon E, Cluzel
P, Grenier P, Rouby JJ. Comparative diagnostic performances of
auscultation, chest radiography and lung ultrasonography in ARDS.
Anesthesiology. 2004;100:9-15.
58. Liu J, Cao HI, Wang HW, Kong XY. Role of lung
ultrasound in diagnosis of respiratory syndrome in newborn infants. Iran
J Pediatr. 2015;25:e323.
59. Raimondi F, Migliaro F, Sodano A, Umbaldo A,
Romano A, Vallone G, et al. Can neonatal lung ultrasound monitor
fluid clearance and predict the need of respiratory support? Crit Care.
2012;16:R220.
60. Raimondi F, Migliaro F, Sodano A, Ferrara T, Lama
S, Vallone G, et al. Use of neonatal chest ultrasound to predict
noninvasive ventilation failure. Pediatrics. 2014;134: e1089-94.
61. Brat R, Yousef N, Klifa R, Reynaud S, Aguilera
SS, De Luca D. Lung ultrasonography score to evaluate oxygenation and
surfactant need in neonates treated with continuous positive airway
pressure. JAMA Pediatr. 2015;169:e151797.
62. Walsh MC, Kliegman RM. Necrotizing enterocolitis:
treatment based on staging criteria. Pediatr Clin North Am.
1986;33:179-201.
63. Faingold R, Daneman A, Tomlinson G,Babyn PS,
Manson DE, Mohanta A, et al. Necrotizing enterocolitis:
assessment of bowel viability with color doppler US. Radiology.
2005;235:587-94.
64. Leonidas JC, Krasna IH, Fox HA, Broder MS.
Peritoneal fluid in necrotizing enterocolitis: a radiologic sign of
clinical deterioration. J Pediatr. 1973;82:672-5.
65. Kim WY, Kim WS, Kim IO, Kwon TH, Chang W, Lee EK,
et al. Sonographic evaluation of neonates with early-stage
necrotizing enterocolitis. Pediatr Radiol. 2005;35:1056-61.
66. Kirks DR, O’Byrne SA. The value of the lateral
abdominal roentgenogram in the diagnosis of neonatal hepatic portal
venous gas (HPVG). Am J Roentgenol Radium Ther Nucl Med. 1974;122:153-8.
67. Silva CT, Danemann A, Navarro OM, Moore AM,
Moineddin R, Gerstle JT, et al. Correlation of sonographic
findings and outcome in necrotizing enterocolitis. Pediatr Radiol.
2007;37:274-82.
68. Garbi Gautel A, Brevaut Malaty V, Panual M,
Michel F, Merrot T, Gire C. Prognostic value of abdominal sonography in
necrotizing enterocolitis of premature infants born before 33 weeks
gestational age. J Pediatr Surg. 2014;49:508-13.
69. Perin G, Scarpa MG. Defining central venous line
position in children: tips for the tip. J Vasc Access. 2015;16:77-86.
70. Jain A, McNamara PJ, Ng E, El-Khuffash A. The use
of targeted neonatal echocardiography to confirm placement of periph-
erally inserted central catheters in neonates. Am J Perinatol.
2012;29:101-6.
71. Tauzin L, Sigur N, Joubert C, Parra J, Hassid S,
Moulies ME. Echocardiography allows more accurate placement of periph-
erally inserted central catheters in low birthweight infants. Acta
Paediatr. 2013;102:703-6.
72. Fleming SE, Kim JH. Ultrasound-guided umbilical
catheter insertion in neonates. J Perinatol. 2011;31:344-9.
73. Stanojevic M. Training of ultrasound in
neonatology: Global or local? Donald School J Ultrasound Obstet Gynecol.
2013;7:338-45.
|
|
 |
|