|
Indian Pediatr 2010;47: 429-432 |
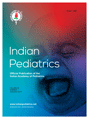 |
Phenotypic Heterogeneity and Parental Origin
of Extra Chromosome 21 in Down Syndrome |
Mamta Muranjan, Tejasvi Chaudhari and*Babu Rao Vundinti
From Genetic Clinic, Department of Pediatrics, KEM
Hospital, Parel, Mumbai 400 012 and *Cytogenetics Division, Institute of
Immunohematology, Indian Council of Medical Research, Mumbai, India.
Correspondence to: Dr Mamta Muranjan, 3rd floor, 301,
Suman Apartments, 16-B, Naushir Bharucha Road, Tardeo, Mumbai 400 007,
India.
Email:
[email protected]
Received: November 23, 2008;
Initial review: December 23, 2008;
Accepted: February 19, 2009.
Published online: May 10, 2009.
PII : S097475590800683-2
|
Abstract
We compared the frequency of phenotypic features of
40 children with Down syndrome between individuals with a maternally or
paternally derived extra chromosome 21, using quantitative FISH for
comparing heteromorphisms of the nucleolar organizing region. Parental
origin was determined in 90% of families. Hypotonia and craniofacial
abnormalities were present in 90% or more individuals, irrespective of
parental origin of chromosome 21. Congenital heart defects were more
frequent in cases with a maternally derived extra chromosome 21.
Imprinted gene(s) may contribute to the development of congenital heart
defects in Down syndrome.
Keywords: Congenital heart disease, Down
syndrome, Fluorescent in-situ hybridization, Genomic imprinting, Trisomy
21.
|
E ighty features are described in
Down syndrome (DS)(1). However, not all features are observed in an
individual with DS. Congenital heart defects (CHD) and duodenal stenosis
are present in only 50% and 7% of cases, respectively(2). Relationship
between extra genes or gene products on chromosome 21 and craniofacial
abnormalities, heart defects, mental retardation and dermatoglyphics has
contributed to the construction of a phenotypic map within the Down
syndrome critical region (DSCR)(3,4). The mechanism for phenotypic
variability has yet not been understood.
Genomic imprinting – a phenomenon where expression of a
gene or a set of genes is determined by the parent of origin, is a
fascinating hypothesis. Features in DS may vary with the parent of origin
of extra chromosome 21. This study aimed at com-paring phenotypic features
of cases with maternal versus paternal origin of extra chromosome 21.
Methods
The study was performed over 10 months after approval
of the Institutional Ethics Committee. We prospectively enrolled 61
patients with Down syndrome. The inclusion criteria were free trisomy 21
confirmed by karyotype and availability of both biological parents. Blood
count, radiographs, ECG, 2D echocardiogram and color doppler, thyroid
function tests (serum T3, T4 and TSH), audiometery or BERA, developmental
quotient, and ophthal-mologic consultation were obtained for all subjects.
Nucleolar organizing region (NOR) heteromorphisms were
studied using quantitative FISH (Q-FISH) by digitally capturing images and
measuring the fluorescent signal intensity. Probes used were FlourX
labeled probe (green fluorescence) hybridizing to the NOR of all
acrocentric chromosomes including chromosome 21 and Cy3 labeled probe (red
fluorescence) hybridizing only to the chromosome 21 specific 21q21.2
region. The probes were synthesized using standard protocols involving
nick translation. The child’s and the parents’ fluorescent NOR signals of
chromosome 21 were quantitated for width, length and fluorescence ratio
using Imstar software (France) and compared. Parent of origin was assigned
on the basis of a match with one parent.
Physical features, congenital anomalies and other
defects were compared between cases with a maternally derived extra
chromosome 21 and those with a paternally derived extra chromosome by
applying Fisher’s test.
Results
Free trisomy 21 was detected in 40 cases (21 males, 19
females). The mean age was 22.1 ± 27.1 months (range: 45 days to 12
years). The mean maternal age and paternal age at delivery was 27 ± 5.6
years (range, 21 to 40 years) and 32.7 ± 7.2 years (range, 24 to 55
years), respectively. Parental origin of chromosome 21 could be determined
in 36 families (90%). The non-dysjunction error was maternal in 77.8% [23
patients (82.1%) with 1st meiotic nondysjunction and 5 (17.9%) with 2nd
meiotic nondysjunction] and paternal in 22.2% [1st meiotic error in 6
cases (75%) and 2nd meiotic error in 2 (25%)]. Maternal 1st meiotic
nondysjunction accounted for 63.9% of the total cases.
Frequency of microcephaly, flat occiput, flat facial
profile, epicanthic folds, oblique palpebral fissures, flat nasal bridge,
open mouth, short neck, curved fifth finger and hypotonia did not differ
significantly with respect to parental origin of chromosome 21. However,
the proportion of children with CHD, high arched palate and short fingers
[94.7% (18/19 cases), 85.7% (24/28 cases) and 71.4% (20/28 cases),
respectively] was significantly greater with maternally derived extra
chromosome 21 as compared to those of paternal origin [16.7% (1/6 cases),
50% (4/8 cases) and 25% (2/8 cases), respectively] (P=0.0006, 0.053
and 0.03, respectively). Peripheral hypoplasia of the iris was more common
when the extra chromosome was paternally derived [37.5% (3/8 cases) versus
3.6% (1/28 cases) of maternal origin, P=0.02].
Discussion
Despite insights into the molecular mechanisms of DS,
there is no convincing explanation for the phenotypic heterogeneity. Given
the role of genomic imprinting in disorders such as Prader-Willi and
Angelman syndromes, it seemed an intriguing hypothesis for the
heterogeneity in DS. Imprinted genes have been identified on mouse
chromosome 16 which is the human homologue of chromosome 21. Henderson,
et al.(5) have postulated the presence of developmentally vital
imprinted genes on chromosome 21. No imprinted genes were identified on
chromosome 21 previously and the role of imprinting in DS was
rejected(6,7). Recently, Luedi, et al.(8) predicted the SIM2
gene as a candidate imprinted gene localizing within the DSCR with
expression of the paternal allele(8). Overexpression of this gene is
implicated in the pathogenesis of mental retardation in DS. This
development prompts re-examination of the role of imprinting in the
pathogenesis of a subset of features in DS.
CHD is the commonest malformation in DS, though it
seldom occurs in mosaic DS(2,9). Previous studies examining the
relationship between parental origin of chromosome 21 and heart defects,
failed to show an association, at least with atrioventricular septal
defects (AVSD)(10). This contrasts with the present study where a
significantly higher proportion of cases with a maternally derived extra
chromosome 21 had CHD, suggesting that one or more imprinted genes on
chromosome 21 may influence the likelihood of developing CHD in DS.
It is now evident that trisomy of genes in the heart
defects critical region are required for development of cardiac defects.
This region contains 64 known or predicted genes. Some of these genes are
expressed in the fetal heart(11,12). It is possible that one or more genes
in the heart defects critical region could be imprinted and that two
copies of the maternally derived genes could tilt the balance in favor of
development of CHD. This study also shows that despite the heterogeneity,
the craniofacial appearance and neurological abnormalities are almost
universal in DS and could be the direct consequence of non-specific
mechanisms of the trisomic state(7).
Lack of access to highly accurate technology using
polymorphic DNA markers to establish parental origin with a success rate
of 96% was a relative disadvantage of the present study. QFQ banding and
silver NOR staining to study heteromorphisms is subjective and identifies
only 54% of cases(13,14). Q-FISH used in the present study yields an
efficiency of 80% to determine chromosome 21 non-dysjunction and
demonstrated a detection rate of 90% in the present study(15).
The number of patients was also less to infer a
definite role for parent of origin in pathogenesis of CHD in DS. It is
also possible that the more frequent origin of the extra chromosome 21
from the mother could be a confounding factor explaining the observations
in the present study(7). Nevertheless the study provides a basis to
examine this phenomenon further.
Acknowledgment
Mrs Seema Korgaonkar, Research Associate, Cytogenetics
Division, Institute of Immuno-hematology, Mumbai, for cytogenetic
preparation and analysis. Dr ME Yeolekar, Dean, Seth GS Medical College
and KEM Hospital, Mumbai for granting permission to publish the paper.
Contributors: MM designed and supervised the study,
interpreted the data and revised the manuscript and will act as guarantor
for the paper. TC collected and analyzed the data and prepared the draft
of the manuscript. VB provided inputs for the methodology and interpreted
the tests.
Funding: None.
Competing interests: None stated.
What This Study Adds?
• Heart defects are more common in Down syndrome individuals
with a maternally derived extra chromosome 21.
|
References
1. Reeves RH, Baxter LL, Richtsmeier JT. Too much of a
good thing: mechanisms of gene action in Down syndrome. Trends Genet 2001;
17: 83-88.
2. Korenberg JR, Bradley C, Distechet CM. Down
Syndrome: Molecular mapping of the congenital heart disease and duodenal
stenosis. Am J Hum Genet 1992; 50: 294-302.
3. Korenberg JR, Chen XN, Schipper R, Sun Z, Gonsky R,
Gerwehr S, et al. Down syndrome phenotypes: The consequences of
chromosomal imbalance. Proc Natl Acad Sci 1994; 91: 4997-5001.
4. Sinet PM, Théophile D. Rahmani Z, et al.
Mapping of the Down syndrome phenotype on chromosome 21 at the molecular
level. Biomed Pharmacother 1994; 48: 247-252.
5. Henderson DJ, Sherman LS, Loughna SC, Bennett PR,
Moore GE. Early embryonic failure associated with uniparental disomy for
human chromosome 21. Hum Mol Genet 1994; 8: 1373 - 1376.
6. Stoll C, Alembik Y, Dott B, Feingold J. No evidence
for genomic imprinting in liveborn Down syndrome patients. Ann Génét 1995;
38: 13-18.
7. Epstein CJ. Down syndrome (Trisomy 21) In: Scriver
CR, Beaudet AL, Valle D, Sly WS (Eds), The Metabolic and Molecular bases
of Inherited Disease. 8 th Edn.
New York: McGraw Hill; 2001. p. 1223-1256.
8. Luedi PP, Dietrich FS, Weidman JR, Bosko JM, Jirtle
RL, Hartemink AJ. Computational and experimental identification of novel
human imprinted genes. Genome Res 2007; 17: 1723-1730.
9. Kohn G, Taysi K, Atkins TE, Mellman WJ. Mosaic
mongolism. Clinical correlation. J Pediatr 1970; 76: 874- 879.
10. Zittergruen MM, Murray JC, Lauer RM, Burns TL,
Sheffield VC. Molecular analysis of non-dysjunction in Down syndrome
patients with and without atrioventricular septal defects. Ciculation
1995; 92: 2803-2810.
11. Maslen CL, Babcock D, Robinson SW, Bean LJH, Dooley
KJ, Willour VL, et al. CRELD1 mutations contribute to the
occurrence of cardiac atrioventricular septal defects in down syndrome. Am
J Med Genet A 2006; 140:2501-2505.
12. Barlow GM, Xiao-Ning C, Shi ZY, Lyons GE, Kurnit
DM, Celle L, et al. Down syndrome congenital heart disease: A
narrowed region and a candidate gene. Genet Med 2001; 3: 91-101.
13. Petersen MB, Frantzen M, Antonarakis SE, Warren AC,
Van Broeckhoven C, Chakravarti A, et al. Comparative study of
microsatellite and cytogenetic markers for detecting the origin of the
nondisjoined chromosome 21 in Down syndrome. Am J Hum Genet 1992; 51:
516-525.
14. Antonarakis SE. Parental origin of the extra
chromo-some in trisomy 21 as indicated by analysis of DNA polymorphisms.
Down Syndrome Collaborative Group. N Engl J Med 1991; 324: 872-886.
15. Iourov IY, Soloviev IV, Vorsanova SG, Monakhov VV, Yurov YB. An
approach for quantitative assessment of fluorescence in situ hybridization
(FISH) signals for applied human molecular cytogenetics. J Histochem
Cytochem 2005; 53: 401-408.
|
|
 |
|