|
Indian Pediatr 2015;52:
1051-1059 |
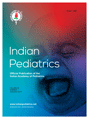 |
Hyperinsulinemic Hypoglycemia in Infancy:
Current Concepts in Diagnosis and Management
|
Shrenik Vora, Suresh Chandran, Victor Samuel
Rajadurai and #Khalid
Hussain
From Department of Neonatology, KK Women’s and
Children’s Hospital, Singapore; and #Genetics and Epigenetics
in Health and Disease Genetics and Genomic Medicine Programme, UCL
Institute of Child Health, Great Ormond Street Hospital for Children, 30
Guilford Street, London, UK.
Correspondence to: Dr Shrenik Vora, Senior Staff
Registrar, Department of Neonatology, KK Women’s and Children’s
Hospital, 100, Bukit Timah Road, Singapore 229899.
Email: [email protected]
|
Purpose: Molecular basis of various forms of hyperinsulinemic
hypoglycemia, involving defects in key genes regulating insulin
secretion, are being increasingly reported. However, the management of
medically unresponsive hyperinsulinism still remains a challenge as
current facilities for genetic diagnosis and appropriate imaging are
limited only to very few centers in the world. We aim to provide an
overview of spectrum of clinical presentation, diagnosis and management
of hyperinsulinism.
Methods: We searched the Cochrane library,
MEDLINE and EMBASE databases, and reference lists of identified studies.
Conclusions: Analysis of blood samples, collected
at the time of hypoglycemic episodes, for intermediary metabolites and
hormones is critical for diagnosis and treatment. Increased awareness
among clinicians about infants "at-risk" of hypoglycemia, and recent
advances in genetic diagnosis have made remarkable contribution to the
diagnosis and management of hyperinsulinism. Newer drugs like lanreotide
(long acting somatostatin analogue) and sirolimus (mammalian target of
rapamycin (mTOR) inhibitor) appears promising as patients with diffuse
disease can be treated successfully without subtotal pancreatectomy,
minimizing the long-term sequelae of diabetes and pancreatic
insufficiency. Newer insights in understanding the molecular and
histological basis and improvements in imaging and surgical techniques
will modify the approach to patients with congenital hyperinsulinism.
Keywords: Congenital hyperinsulinism, Diazoxide,
Insulin-secreting cells
|
Hyperinsulinemic hypoglycemia has increasingly
been recognized as a cause of intractable hypoglycemia in neonates and
infants. Hyperinsulinemic hypoglycemia occurs due to unregulated insulin
secretion from b-cells
of pancreas in relation to blood glucose levels [1]. Small for
gestational age (SGA) infants and macrosomic infants born to diabetic
mothers (IDM) are the two most common groups of infants at risk of
hypoglycemia in the neonatal period [2]. Glucose is the principal energy
source for the neonatal brain and hypoglycemia is known to cause
irreversible neuronal injury when it is recurrent and severe; so prompt
recognition and treatment of these infants with hyperinsulinemic
hypoglycemia is paramount [3]. Hyperinsulinemic hypoglycemia can be
transient, prolonged or persistent (congenital). Knowledge of blood
glucose homeostasis and appropriate investigations for intermediary
metabolites during an episode of hypoglycemia is the cornerstone for
diagnosis and management of hyperinsulinemic hypoglycemia. The
management of medically unresponsive hyperinsulinemic hypoglycemia still
remains a challenge. Knowledge of the genetic mutations, newer imaging
modalities like Fluorine 18L-3, 4-dihydroxyphenylalanine positron
emission tomography (18F-DOPA-PET)
scan and availability of histological differentiation of focal and
diffuse forms of persistent hyperinsulinemic hypoglycemia has
streamlined the management of congenital hyperinsulinemic hypoglycemia
(CHI) [4]. The purpose of this review is to provide an overview of
physiology of insulin secretion, controversies regarding definition of
hypoglycemia, spectrum of clinical presentation, and recent advances in
diagnosis and management of different forms of hyperinsulinism.
Glucose Homeostasis
Normoglycemia is maintained by a balance between the
insulin secretion following food intake and response of the counter
regulatory hormones (glucagon, growth hormone, adrenaline and cortisol)
in the face of hypoglycemia. Insulin promotes peripheral uptake of
glucose and counter regulatory hormones increase hepatic glucose output
by glycogenolysis followed by gluconeogenesis. Liver glycogen stores in
a healthy full-term infant last for 10-12 hours from birth. Endocrine
changes occur soon after birth with a decrease in plasma levels of
insulin and increase of catecholamine and glucagon [5]. Hepatic glucose
production in a full-term infant is 4-6 mg/kg/min and the infant
switches on the endogenous production of glucose following birth until
exogenous nutritional supply is established. The newborn infant has
essential enzymes needed for gluconeogenesis from alanine, pyruvate,
glycerol, and lactate. Soon after birth hepatic glycogenolysis provides
glucose whereas b-oxidation
of fatty acids and lactate generation due to proteolysis provide an
adequate substrate for gluconeogenesis [6]. Infants with disorders of
glycogenolysis present after 4-5 hours of fast whereas infants with
disorders of gluconeogenesis become hypoglycemic after an overnight
fast. On the contrary, infants with hyperinsulinism present with
hypoglycemia at any time after the last feed [7].
Insulin Release from
b-cells of Pancreas
Knowledge of insulin secretion by
b-cells of pancreas
in response to plasma glucose helps to understand the pathogenesis and
management of hyperinsulinemic hypoglycemia. As shown in Fig.1,
the metabolism of glucose, amino acids and fatty acids results in the
generation of metabolic coupling factors like ATP and
b-ketoglutarate,
which are involved in regulating insulin exocytosis. Under normal
physiological conditions, each of these coupling factors plays a key
role in regulating insulin secretion precisely to keep fasting blood
glucose concentrations between 3.5-5.9 mmol/L. As blood glucose level
rises after feed or glucose infusion, it triggers insulin secretion from
pancreatic b-cells.
The GLUT2 transporters present on pancreatic
b-cells facilitate
the uptake of glucose. Glycolytic phosphorylation by glucokinase (GK)
enzyme follows leading to a rise in the ATP:ADP ratio. Functional
integrity of the pancreatic ATP sensitive potassium (KATP)
channel depends on the interactions between the pore-forming inward
rectifier potassium channel subunit (KIR6.2) and the regulatory
sulfonylurea receptor 1(SUR1), encoded by KCNJ11 and ABCC8
genes, respectively. The increase in the cytosolic ATP: ADP ratio
activates plasma membrane SUR1, which leads to the closure of KATP channel. This in turn depolarizes the membrane
allowing calcium ions to flow in to the cell via voltage-gated calcium
channels. Following this, the insulin storage granules undergo
exocytosis resulting in release of insulin [8]. Insulin secretion is
also regulated by metabolic signals arising from lipid and amino acid
metabolism mediated through its effect on glutamate dehydrogenase (GDH)
[9].
Definition of Hypoglycemia
Definition of hypoglycemia and screening guidelines
in neonatal period remain controversial. Following severance of the
umbilical cord at birth, glucose supply from the mother to the neonate
ceases leading to a rapid fall in neonatal glucose concentration.
Glucose level decreases reaching a nadir at 1 hour of age and then
stabilizes by 3 hours of age spontaneously or in response to milk feeds
in healthy full-term infants.
In a comprehensive review of the literature in 1997,
an expert panel of the World Health Organization concluded that there
are numerous approaches to defining normoglycemia, including the
statistical, metabolic, neurophysiological, and, perhaps most
importantly, the neurodevelopmental approach. These different approaches
towards definition of normoglycemia contribute to the controversy that
surrounds this issue [10]. Current consensus by world experts on
hyperinsulinism states that hypoglycemia cannot be defined as a specific
plasma glucose concentration due to inability to identify a single value
that causes brain damage and brain responses varies by the presence of
alternative fuels like ketones [11]. Currently the most common practice
is to screen the at-risk infants, which includes IDM and neonatal
conditions like SGA (birth weight <10 th
centile), large for gestational age (LGA, birth weight >90th
centile), perinatal asphyxia, prematurity, infection, and dysmorphic
infants suggestive of Beckwith-Wiedemann syndrome. This has led to the
development of guidelines designed to identify infants "at-risk" and the
implementation of an "operational threshold" for physicians to consider
intervention [12].
In 2011, clinical report of the American Academy of
Pediatrics recommended screening of "at-risk" infants within first hours
of birth. The macrosomic IDM, late-preterms (34 to 36 +6
weeks gestation) and SGA infants should be fed every 2-3 hours with
estimation of pre-feed glucose levels for multiple feed-fast cycles for
at least 24 hours. Further monitoring of blood sugar levels should be
continued only if plasma glucose levels remain below 2.5 mmol/L.
Symptoms in infants with blood glucose value less than 2.2 mmol/L
warrant parenteral glucose infusion. In asymptomatic infants, a
practical approach based on age, risk factors and mode of feeding can be
considered when treatment is planned. Any infant with persistent or
recurrent hypoglycemia should be screened for hyperinsulinism [13].
Hyperinsulinemic hypoglycemia is defined as inappropriately elevated
plasma insulin concentration in the presence of hypoglycemia (<3.5
mmol/L) in infants receiving glucose infusion rate (GIR) of more than
8mg/kg/min, with suppressed ketone bodies and free fatty acids and a
positive glycemic response to parenteral glucagon. In infants with
suspected hyperinsulinemic hypoglycemia, due to lack of alternative
energy fuels the blood glucose levels should be maintained >3.5 mmol/L
[14].
Types of Hyperinsulinemic Hypoglycemia
Hyperinsulinemic hypoglycemia can be transient,
prolonged or persistent (congenital).
Transient Hyperinsulinemic Hypoglycemia
This is observed often in IDM, SGA infants and in
infants who had perinatal asphyxia, polycythemia and Rh isoimmunization.
There is no clear definition of the precise duration of transient
hypoglycemia. Hyperinsulinemic hypoglycemia usually presents soon after
the birth and settles within a few days responding to increment of feeds
or to increasing glucose infusion rate until the
b-cell insulin
secretion is normalized [15].
Prolonged Hyperinsulinemic Hypoglycemia
Some of the SGA infants develop a syndrome of
prolonged hyperinsulinemic hypoglycemia requiring high GIR to maintain
normoglycemia and responding to medical treatment with K ATP
channel agonist (diazoxide). The etiology of prolonged hyperinsulinemic
hypoglycemia in SGA infants could be due to a lack of supply of
exogenous substrate, depletion of hepatic glycogen stores, defective
gluconeogenesis, hyper-insulinism due to transient alteration in the
regulation of b-cell
insulin secretion, increased sensitivity to insulin or adrenocortical
insufficiency. No genetic cause has been identified in prolonged
hyperinsulinemic hypoglycemia and in most of the cases, resolution is
observed in several weeks to months. The incidence of prolonged
hyperinsulinemic hypoglycemia is reported to be as high as 1:12,000
births. There are few reports where the prolonged hyperinsulinemic
hypoglycemia was diagnosed as late as 2 weeks after birth (range: 2-180
days) and hence there is a risk involved in early discharge of these SGA
infants [16].
Congenital Hyperinsulinism (CHI)
CHI is a heterogeneous condition presenting with
hyperinsulinism, hypoketonemia, and hypo-fattyacidemia with severe and
persistent hypoglycemia. The etiopathogenesis of CHI can be due to two
major defects known as channelopathies and metabolopathies.
Channelopathies refer to defects in the pancreatic â-cell ATP-sensitive
K ATP channel that lead to
unregulated insulin secretion, the commonest genetic cause being
autosomal recessively inherited inactivating mutation in ABCC8
and KCNJ11(chromosome11p15.1) genes [17]. Metabolopathies cause
congenital hyperinsulinemic hypoglycemia either by altering the
concentration of intracellular signaling molecules (such as ATP/ADP) or
by accumulation of intermediary metabolites, triggering insulin release.
The commonest cause for metabolopathies is
Hyperinsulinism-Hyperammonemia (HI/HA) syndrome. The incidence of CHI is
estimated to be 1:40,000-50,000 in the general population, but in
familial forms it may be as high as 1:2500 in populations with
substantial consanguinity [18].
Genetics of congenital hyperinsulinism
Genetic defects in key genes regulating insulin
secretion causes CHI. Mutations in nine genes – ABCC8, KCNJ11, GLUD1,
GCK, HADH, SLC16A1, HNF4A, HNF1A, UCP2 – have been identified to
cause CHI, altering the b-cell
function [19].
Histologically, focal and diffuse forms have been
reported. Diffuse forms are inherited in an autosomal recessive or
dominant manner and focal forms are sporadic in nature.
CHI due to channelopathies: Recessive
inactivating mutations in ABCC8 and KCNJ11 genes
are the most common causes of CHI and these mutations are found in 50%
of the patients. These mutations alter the function of K ATP
channel, causing unregulated insulin secretion. This results in severe
CHI and is unresponsive to KATP
channel agonist, diazoxide. Histologically, recessive KATP
channel mutation is characterized by large
b-cells with enlarged
nuclei. A milder form of CHI has been reported with dominant
inactivating mutations in ABCC8 and KCNJ11 genes. These
milder forms are also reported to be mostly unresponsive to diazoxide.
In ABCC8 gene about 150 homozygous, compound heterozygous and
heterozygous inactivating mutations and in KCNJ11 around 24
mutations have been reported [20].
Focal lesions due to K ATP
mutations are confined to restricted areas of pancreas. Chromosome
11p15.5 (in close proximity to KATP
channel gene 11p15.1) has maternally expressed tumor suppressors H19
and CDK1C and paternally expressed growth factor gene,
IGF2. Genetically focal lesion arises following a paternally
inherited, monoallelic mutation in one of the KATP
channel genes. During the development of pancreas, when segmental
paternal uniparental disomy occurs as a somatic mutation, KATP
channel activity is lost in the b
cell. These cells also lose maternally expressed tumor suppressor
activities of H19 and CDK1C and the activity of paternally
expressed IGF2 is doubled, promoting the growth of abnormal
b-cells.
Histology reveals focal enlargement of
b-cell with large
nuclei. Generally (96.2%) focal lesions are unresponsive to diazoxide
but are curable by limited excision [7, 18, 21].
HI/HA syndrome: It is the second commonest form
of CHI, caused by activating missense mutation of the GLUD1 gene, which
encodes the mitochondrial enzyme GDH. GDH is expressed in pancreatic
b-cells,
liver, kidney and brain. GLUD1 gene mutations lead to a gain of GDH
function by reducing its sensitivity to allosteric inhibition by GTP and
ATP, resulting in activation of insulin secretion by the amino acid
leucine. Mechanism of hyperammonemia in HI/HA syndrome is unclear, may
be due to increased hepatic GDH activity and ammonia synthesis or due to
abnormal muscle catabolism. Recently, renal ammoniagenesis has been
implicated as a source of HA [22]. Hyperammonemia, a characteristic
biochemical marker of HI/HA syndrome, is typically mild to moderate in
infants and is not associated with lethargy or coma. Some of these
patients may not have hyperammonemia and it could be due to mosaicism
for the mutation in the liver, where the mutation is absent or seen in
<50% in hepatocytes. Infants with HI/HA syndrome experience both fasting
and postprandial hypoglycemia following leucine intake usually after
first few months of life. Diazoxide remains the mainstay of treatment in
patients with HI/HA syndrome [23].
Hydroxyacyl Coenzyme A Dehydrogenase (HADH) gene
mutation: Short-chain-3-hydroxyacyl-CoA dehydro-genase (SCHAD),
encoded by the gene HADH, catalyses the penultimate reaction of the
b-oxidation
cycle [24]. HADH is expressed in pancreatic
b-cells, indicating a
vital role in insulin secretion. In patients with HADH gene mutation,
"protein to protein" interaction is lost between GDH and SCHAD causing
leucine-induced hyperinsulinemic hypoglycemia via a novel pathway not
involving GTP regulation of GDH. The clinical phenotype of HADH
mutations varies from mild to severe neonatal hyperinsulinemic
hypoglycemia with raised levels of fatty acid metabolites responding to
diazoxide [25].
Glucokinase – Cytosolic enzyme defects:
Glucokinase (GCK) is a glycolytic enzyme and plays an important role
as a glucose sensor in the pancreatic
b-cell controlling
glucose regulated insulin secretion. Heterozygous activating mutations
have been reported to cause CHI and inactivating monoallelic mutations
causing mild form of diabetes (GCK-MODY). Inappropriate insulin
secretion following heterozygous activating mutations of glucokinase
resulted from increased affinity of the enzyme for glucose, raising the
ATP: ADP ratio in the b-cell
[26]. Affected patients present with fasting hypoglycemia and can be
symptomatic anytime from infancy to adulthood. Mostly this form of
hyperinsulinemic hypoglycemia is diazoxide-responsive but cases
requiring octreotide and subtotal pancreatec-tomy have been reported.
Mutations in mitochondrial uncoupling protein 2
(UCP2) gene: UCP2 acts as a negative regulator of insulin
secretion of b-cells.
It has been shown that UCP2 uncouples mitochondrial oxidative
phosphorylation from ATP generation. Loss of function mutation of
UCP2 gene has been reported to cause transient hyperinsulinemic
hypoglycemia or mild fasting diazoxide responsive CHI [26, 27].
HNF4A/HNF1A gene mutations: HNF4A
gene encodes for a transcription factor HNF4 a
(hepatocyte nuclear factor 4a),
a member of the nuclear hormone receptor superfamily. Mutations in
HNF4A gene are uncommon causes of hyperinsulinemic
hypoglycemia, which can be either transient or persistent. HNF4A
is required in the pancreatic b-cell
for the regulation of the pathway of insulin secretion, heterozygous
mutations of which cause maturity-onset diabetes of the young type
1(MODY1) [28]. Exact mechanism of hyperinsulinemic hypo-glycemia due to
HNF4A gene mutations is unclear. The possible mechanisms might be
a reduction in expression of the potassium channel subunit (Kir6.2) or
reduction in expression of nuclear PPARa
(peroxisome proliferator-activated receptor
a), which can shift
energy metabolism in cells towards fatty acid oxidation (FAO). Similar
to HNF4A, HNF1A mutations can cause hyperinsulinism in
newborn and infancy, and diabetes in later life [29].
Exercise-induced hyperinsulinemic hypoglycemia
(SLC16A1 gene mutation): This is a dominantly inherited form of CHI
characterized by inappropriate insulin secretion following anaerobic
exercise or pyruvate load. In normal physiological status, lactate and
pyruvate transport into the a-cell
is mediated by monocarboxylase transporter 1(MCT1), which is encoded by
the SLC16A1 gene. Under normal circumstances, MCT1 expression in
the pancreatic a-cell
is very low, minimizing the effects of pyruvate and lactate on insulin
secretion. Increased levels of MCT1 due to promoter-activating mutations
in SLC16A1 gene permits entry of circulating lactate and pyruvate
into the b-cell,
leading to an increase in ATP generation, triggering insulin release by
closure of KATP channel and
depolarization of the cell. Affected children become hypoglycemic
typically 30-45 minute after a period of intensive anaerobic exercise
due to lactate and pyruvate accumulation [19,30]. Exercise-induced
hyperinsulinemic hypoglycemia is not observed in neonates and reported
cases are limited to older children and adults.
Other causes of hyperinsulinemic hypoglycemia:
Rarely, hyperinsulinemic hypoglycemia is also seen associated with
overgrowth syndromes like Beckwith-Wiedemann and Sotos syndromes and
metabolic conditions like congenital disorder of glycosylation (CDG) and
tyrosinemia. Beckwith-Wiedemann syndrome, the most common syndrome
associated with hyperinsulinemic hypoglycemia is characterized by
overgrowth, macroglossia, hemi-hypertrophy and abdominal wall defects.
The hypoglycemia can be asymptomatic transient to rarely prolonged,
extending beyond neonatal period [31]. CDG type Ib
(phosphomannose-isomerase deficiency), perhaps causing abnormal
glycosylation of K ATP
channel, causes hyperinsulinism and that may explain the response of
hyperinsulinemic hypoglycemia in these infants to diazoxide [32]. The
mechanism of hyperinsulinemic hypoglycemia in tyrosinemia type 1 is
still unclear but may be due to accumulation of toxic metabolites
causing islet-cell hyperplasia [33].
Clinical Presentation
Patients with hyperinsulinemic hypoglycemia present
during the newborn period most often during the first 24-48 hours of
life. However, different studies showed median age of presentation
ranging from hours to weeks. Symptoms of hypoglycemia are mostly
non-specific such as lethargy, poor feeding, apnea, jitteriness,
irritability, high-pitched cry, exaggerated reflexes, seizures and coma.
Presentation of persistent hyperinsulinemic hypoglycemia is more severe
needing higher concentrations of glucose to maintain the blood glucose
level [34]. High index of suspicion, early diagnosis and aggressive
management is essential to prevent unexplained deaths and brain injury
due to hypoglycemia.
Neonatal Hypoglycemic Brain Injury (NHBI):
Hypoglycemia, being a surrogate marker of neuronal energy deficiency, is
a major cause of brain injury. Speculated mechanisms of cellular injury
includes; excitatory neurotoxins active at N-methyl-D-aspartate
receptors, increased mitochondrial free radical generation and
initiation of apoptosis. Several neuroprotective mechanisms are believed
to play a role to guard against these neuronal injuries by substitution
of alternative cerebral substrates like lactate, ketone bodies,
pyruvate, amino acids, free fatty acids and glycerol [35]. It has been
postulated that immature newborn brain requires decreased cerebral
energy fuels as compared to children and adults. Also limited glycogen
stores in astrocytes provide immediate supply of glucose to the neurons.
These mechanisms can protect the brain for limited periods and permanent
damage occurs if recurrent protracted hypoglycemia prevails [36]. An
increased risk of cerebral palsy, development delay and low mental
scores at 18 months of age has been shown in infants with recurrent
hypoglycemia lasting for five or more days. Pathological changes of NHBI
include swelling of the neuronal and glial cells, necrosis, gyrus
atrophy and white matter demyelination [37]. Neonatal hypoglycemic brain
injury predominantly affects parieto-occipital regions as evidenced by
MRI scans [38].
Diagnosis
A detailed history related to pregnancy
(diabetes/diet/insulin), delivery (asphyxia), gestational age and birth
weight (SGA/LGA/macrosomia) is essential. Parental consanguinity, family
history of diabetes, and history of siblings having infantile seizures
may indicate inherited cause of the hypoglycemia. A thorough physical
examination of the infant to look for dysmorphic features (e.g.
omphalocele, hemihypertrophy, macroglossia), evidence of hypopituitarism
(e.g. cleft lip/palate, micropenis, short stature), adrenal
insufficiency (e.g. hyperpigmentation, weight loss) and disorders of
glycogenesis (e.g. hepatosplenomegaly) has to be carried out. Sudden
cardio-respiratory arrest and acidosis with hypoglycemia in an otherwise
healthy infant might point towards a metabolic disorder [39]. As
mentioned previously, "at-risk" groups of infants should be screened for
hypoglycemia in relation to the risk factors specific to the individual
case. Any patient with recurrent or persistent hypoglycemia despite GIR
of >8mg/kg/min is indicative of hyperinsulinemic hypoglycemia. The key
feature of hyperinsulinemic hypoglycemia is detectable serum insulin
and/or C-peptide levels during episodes of hypoglycemia along with
hypoketonemia and hypofattyacidemia. When diagnosis is in doubt, a
positive glycemic (>1.5mmol/L) response to glucagon or octreotide
therapy gives a supportive evidence of hyperinsulinemic hypoglycemia
[40]. Harris, et al. [41] showed a good correlation between
continuous interstitial glucose monitoring and blood glucose
measurements. Reports suggest that continuous interstitial glucose
monitoring can potentially be advantageous in measuring the duration,
severity, and frequency of low glucose concentrations in high-risk
infants and can help identify and prevent unwanted periods of
hypoglycemia or hyperglycemia [42]. To aid in the diagnosis, further
assessment of plasma and urine metabolic profile (Box 1)
and genetic testing need to be done in some infants presenting with more
subtle forms of CHI, after liaising with local tertiary centres [43].
Additional provocation tests (leucine / protein loading or exercise
testing) are indicated in those patients with protein/leucine
sensitivity and exercise-induced hypoglycemia.
BOX 1 Investigations for Suspected Hyperinsulinemic Hypoglycemia
Urine |
Blood |
Reducing substances |
Glucose |
Ketone bodies |
Insulin / C-peptide |
Amino acids |
Ketone bodies |
Organic acids |
Free fatty acids |
|
Amino acids |
|
Lactate |
|
Ammonia |
|
Bicarbonate |
|
Blood gas analysis |
|
Inborn error of metabolism(IEM) screen |
|
Acyl carnitine profile |
|
Cortisol |
|
Growth hormone |
|
Insulin Growth Factor Binding Protein 1 (IGFBP1) |
Management of Hyperinsulinemic Hypoglycemia
The management of patients with hyperinsulinemic
hypoglycemia can be extremely complicated, particularly in prolonged and
persistent hyperinsulinemic hypo-glycemia. They will require frequent
blood glucose monitoring and the insertion of a central venous catheter
to deliver concentrated dextrose infusion. Ideally, these patients
should be managed at specialized centers that have the necessary
multidisciplinary team experience and expertise [44]. Expert review
suggests that the goal of treatment for "at-risk" infants without
suspected CHI should be to maintain blood glucose levels >2.8 mmol/L for
those aged <48 hours and >3.3 mmol/L for those aged >48 hours. For
neonates with suspected CHI, recommendation is to keep blood glucose
levels >3.9 mmol/L [11].
The treatment of hyperinsulinemic hypoglycemia
involves medical therapy, and surgery in some cases. The mainstay of
initial medical treatment is the provision of adequate carbohydrate to
maintain normoglycemia i.e. blood sugar level between 3.5-6 mmol/L.
Sometimes, to ensure regular frequent feeds, feeding via naso-gastric
tube or feeding gastrostomy may be needed. Symptomatic hypoglycemia is
treated with "minibolus" of intravenous 10% dextrose at 2 mL/kg to
achieve normoglycemia and higher dextrose concentrations should be
avoided as bolus to prevent rebound hypoglycemia by further stimulating
insulin secretion. This is followed by gradually increasing GIR
depending on blood sugar levels by using intravenous glucose at high
concentrations [45].
Diazoxide, a K ATP
channel agonist, is the mainstay of medical treatment in
prolonged hyperinsulinemic hypoglycemia. It prevents
a-cell membrane
depolari-zation and inhibits insulin secretion by keeping KATP
channels open. It is given orally in the dose of 5-20 mg/kg/day in 3
divided doses. When using diazoxide in infants having hepatic
dysfunction or hypoalbuminemia, use lower doses of 3 mg/kg/day as it is
highly protein bound (>90%). Also lower doses are safer in SGA infants
as they are very sensitive to diazoxide [46]. Diazoxide is usually
combined with thiazide diuretics to counteract its most common side
effect of fluid retention in neonates [1]. Hypertrichosis is another
important common complication of diazoxide which usually reverts
following cessation of therapy. Diazoxide responsiveness is noted when
infant can fast appropriately for age, maintains normal glucose levels
and shows rise in serum fatty acids and ketone bodies at the end of fast
[36,40]. Nifedipine, a calcium channel blocker, has been used in few
diazoxide unresponsive cases of CHI, but vast majority of such patients
fail to show any response [47].
Octreotide, a somatostatin analogue causing
inhibition of insulin release by activation of somatostatin receptor-5,
stabilizing K ATP channel and
inhibition of calcium mobilisation is used in resistant cases. It is
given as 6-8 hourly subcutaneous injections in a dose of 5-25 mcg/kg/day
[48]. Recently, few articles suggest once-a-month intramuscular
treatment with long-acting release octreotide (Lanreotide) as a simple,
cost-effective and efficient alternative to thrice daily octreotide
[49]. Glucagon is primarily used for management of acute symptomatic
hypoglycemia as subcutaneous injection at dose of 0.5-1 mg in cases
where intravenous access is difficult. It is also used as continuous
intravenous infusion at dose of 1-20 mcg/kg/hour for short-term
stabilization of glucose control in combination with octreotide in
patients with hyperinsulinemic hypoglycemia. It activates adenylate
cyclase via G-protein-coupled receptor thereby increasing glycogenolysis
and gluconeogenesis [50]. Recent advances have shown the effectiveness
of the mammalian target of rapamycin (mTOR) inhibitor, Sirolimus in
infants with severe diffuse form of hyperinsulinemic hypoglycemia that
had been unresponsive to maximal doses of diazoxide and octreotide [51].
Once glucose levels are stabilized, reduce and stop intravenous glucose
infusion followed by glucagon and octreotide. The maintenance dose of
octreotide is reduced in patients with severe hepatic impairment. These
children with CHI are always at increased risk of infection due to
central line related sepsis and prolonged/multiple hospitalizations.
Molecular testing for mutations in genes responsible
for CHI becomes necessary if hypoglycemia is diazoxide-unresponsive
[52]. Confirmed cases of CHI should be differentiated into focal and
diffuse forms using 18F-DOPA-PET
scan to make surgical decision [53]. Laparoscopic excision is curative
in focal forms whereas near total pancreatectomy is needed in diffuse
disease [54]. Decision for surgery also includes dependence on high GIR
requirement along with unresponsiveness to medications. In immediate
post-operative period, some children may develop transient hyperglycemia
needing insulin administration. Many children have persistent
hypoglycemia following surgery needing on-going diazoxide therapy,
before developing diabetes later on. Near total pancreatectomy leads to
post-operative diabetes and exocrine pancreatic insufficiency in most of
the infants with diffuse persistent hyperinsulinemic hypoglycemia [55].
Infants on treatment for CHI should have long-term developmental follow
up due to high risk of neurodevelopmental delay, cerebral palsy and
epilepsy [35,36]. All the familial forms of CHI should undergo genetic
counseling. Fig. 2 suggests outline for the diagnosis and
management of hyperinsulinemic hypoglycemia.
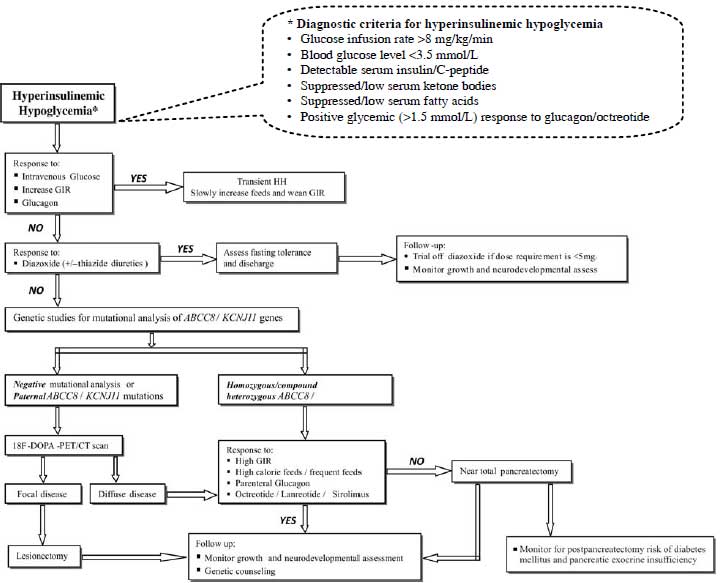 |
Fig. 2 Suggested outline for diagnosis
and management of hyperinsulinemic hypoglycemia.
|
Conclusion
Being an important cause of hypoglycemia in infancy,
early diagnosis and aggressive management of hyperinsulinemic
hypoglycemia is the cornerstone for prevention of hypoglycemia induced
neuronal injury. Molecular basis of the various forms of
hyperinsulinemic hypoglycemia involving defects in key genes, which
regulate insulin secretion, are beginning to be understood and being
increasingly reported. One should keep in mind, the spectrum of clinical
presentations of hyperinsulinemic hypoglycemia. Diazoxide
unres-ponsiveness in a baby with hyperinsulinemic hypoglycemia warrants
genetic studies to look for common mutations and trials with newer drugs
like lanreotide and sirolimus appears promising. However, the management
of hyperinsulinemic hypoglycemia still remains a challenge to the
neonatologists and endocrinologists, even in developed countries; due to
lack of facilities for genetic studies and 18F-DOPA-PET
scan. Novel insights in identifying genetic mechanisms in CHI will
modify the futuristic approach to diagnosis and treatment of
hyperinsulinemic hypoglycemia.
Contributors: SV: Literature search, manuscript
drafting, review and editing; SC: Literature review and manuscript
editing; VSR: Literature review and manuscript editing; KH: Guidance and
final editing of manuscript with extensive literature search. All
authors approved the final manuscript. SV will be the guarantor.
Funding: None; Competing interest:
None stated.
References
1. Arya VB, Senniappan S, Guemes M, Hussain K.
Neonatal hypoglycemia. Indian J Pediatr. 2014; 81:58-65.
2. Straussman S, Levitsky LL. Neonatal hypoglycemia.
Curr Opin Endocrinol Diabetes Obes. 2010;17:20-4.
3. Menni F, de Lonlay P, Sevin C, Touati G, Peigne´
C, Barbier V, et al. Neurologic outcomes of 90 neonates and
infants with persistent hyperinsulinemic hypoglycemia. Pediatrics.
2001;107:476-9.
4. Hussain K, Blankenstein O, De Lonlay P, Christesen
HT. Hyperinsulinaemic hypoglycaemia: biochemical basis and the
importance of maintaining normoglycaemia during management. Arch Dis
Child. 2007;92:568-70.
5. Hawdon J. Glucose homeostasis in the healthy fetus
and neonate. In: Rennie JM, editor. Metabolic and Endocrine
Disorders. Textbook of Neonatology. London: Elsevier, Churchill
Livingstone. 2005;851-52.
6. Henquin JC. Triggering and amplifying pathways of
regulation of insulin secretion by glucose. Diabetes. 2000;49:1751-60.
7. Yorifuji T. Congenital hyperinsulinism: Current
status and future prospectives. Ann Pediatr Endocrinol Metab.
2014;19:57-68.
8. Inagaki N, Gonoi T, Clement JP, Namba N, Inazawa
J, Gonzalez G, et al. Reconstitution of IKATP: An inward
rectifier subunit plus the sulfonylurea receptor. Science.
1995;270:1166-70.
9. Zhang T, Li C. Mechanisms of amino acid-stimulated
insulin secretion in congenital hyperinsulinism. Acta Biochim Biophys
Sin (Shanghai). 2013;45:36-43.
10. World Health Organization. Hypoglycaemia of the
Newborn. Review of the Literature. Geneva: WHO, 1997;WHO/CHD/97.1.
11. Thornton PS, Stanley CA, De Leon D, Harris D,
Haymond MW, Hussain K, et al. Recommendations from the Pediatric
Endocrine Society for Evaluation and Management of Persistent
Hypoglycemia in Neonates, Infants, and Children. J Pediatr. 2015;
167:238-45.
12. Tin W. Defining neonatal hypoglycaemia: a
continuing debate. Semin Fetal Neonatal Med. 2014;19:27-32.
13. Adamkin DH, Committee on Fetus and Newborn.
Postnatal glucose homeostasis in late-preterm and term infants.
Pediatrics. 2011;127:575-79.
14. Kapoor RR, Flanagan SE, James C, Shield J, Ellard
S, Hussain K. Hyperinsulinaemic hypoglycaemia. Arch Dis Child.
2009;94:450-7.
15. Vanhaltren K, Malhotra A. Characteristics of
infants at risk of hypoglycaemia secondary to being ‘infant of a
diabetic mother’. J Pediatr Endocrinol Metab. 2013;26:861-5.
16. Chong JH, Chandran S, Agarwal P, Rajadurai VS.
Delayed presentation of prolonged hyperinsulinaemic hypogly-caemia in a
preterm small-for-gestational age neonate. BMJ Case Rep. 2013.
Doi:10.1136/bcr-2013-200920.
17. Bellanne´-Chantelot C, Saint-Martin C, Ribeiro
MJ, Vaury C, Verkarre V, Arnoux JB, et al. ABCC8 and KCNJ11
molecular spectrum of 109 patients with diazoxide-unresponsive
congenital hyperinsulinism. J Med Genet. 2010;47:752–59.
18. James C, Kapoor RR, Ismail D, Hussain K. The
genetic basis of congenital hyperinsulinism. J Med Genet.
2009;46:289-99.
19. Mohammed Z, Hussain K. The genetics of
hyperinsulinemic hypoglycemia. NeoReviews. 2013;14: 179-88.
20. Verkarre V, Fournet JC, de Lonlay P, Gross-Morand
MS, Devillers M, Rahier J, et al. Paternal mutation of the
sulfonylurea receptor (SUR1) gene and maternal loss of 11p15 imprinted
genes lead to persistent hyperinsulinism in focal adenomatous
hyperplasia. J Clin Invest. 1998;102:1286-91.
21. Flanagan SE, Clauin S, Bellanne´-Chantelot C, de
Lonlay P, Harries LW, Gloyn AL, et al. Update of mutations in the
genes encoding the pancreatic b-cell K (ATP) channel subunits Kir6.2
(KCNJ11) and sulfonylurea receptor 1 (ABCC8) in diabetes mellitus and
hyperinsulinism. Hum Mutat. 2009;30:170-80.
22. Stanley CA, Lieu YK, Hsu BY, Burlina AB,
Greenberg CR, Hopwood NJ, et al. Hyperinsulinism and
hyperammonemia in infants with regulatory mutations of the glutamate
dehydrogenase gene. N Engl J Med. 1998;338:1352-7.
23. MacMullen C, Fang J, Hsu BY, Kelly A, de
Lonlay-Debeney P, Saudubray JM, et al. Hyperinsulinism/hyperammonemia
syndrome in children with regulatory mutations in the inhibitory
guanosine triphosphate-binding domain of glutamate dehydrogenase. J Clin
Endocrinol Metab. 2001;86:1782-7.
24. Hussain K, Clayton PT, Krywawych S, Chatziandreou
I, Mills P, Ginbey DW, et al. Hyperinsulinism of infancy
associated with a novel splice site mutation in the SCHAD gene. J
Pediatr. 2005;146:706-8.
25. Chandran S, Yap F, Hussain K. Molecular
mechanisms of protein induced hyperinsulinaemic hypoglycaemia. World J
Diabetes. 2014;5:666-77.
26. Cuesta-Muńoz A, Huopio H, Otonkoski T, Gomez-Zumaquero
JM, Näntö-Salonen K, Rahier J, et al. Severe persistent
hyperinsulinemic hypoglycemia due to a de novo glucokinase mutation.
Diabetes. 2004;53:2164-8.
27. Gonzalez Barroso MM, Giurgea I, Bouillaud F,
Anedda A, Bellanne Chantelot C, Hubert L, et al. Mutations in
UCP2 in congenital hyperinsulinism reveal a role for regulation of
insulin secretion. PLoS One. 2008;3:e3850.
28. Gupta RK, Vatamaniuk MZ, Lee CS, Flaschen RC,
Fulmer JT, Matschinsky FM, et al. The MODY1 gene HNF 4alpha
regulates selected genes involved in insulin secretion. J Clin Invest.
2005;115:1006 15.
29. Pearson ER, Boj SF, Steele AM, Barrett T, Stals
K, Shield JP, et al. Macrosomia and hyperinsulinaemic hypo-glycaemia
in patients with heterozygous mutations in the HNF4A gene. PLoS Med.
2007;4:e118.
30. Otonkoski T, Kaminen N, Ustinov J, Lapatto R,
Meissner T, Mayatepek E, et al. Physical exercise-induced
hyperinsulinemic hypoglycemia is an autosomal-dominant trait
characterized by abnormal pyruvate induced insulin release. Diabetes.
2003;52:199-204.
31. Munns CF, Batch JA. Hyperinsulinism and Beckwith-Wiedemann
syndrome. Arch Dis Child Fetal Neonatal Ed. 2001;84:F67–9.
32. Sun L, Eklund EA, Chung WK, Wang C, Cohen J,
Freeze HH. Congenital disorder of glycosylation id presenting with
hyperinsulinemic hypoglycemia and islet cell hyperplasia. J Clin
Endocrinol Metab. 2005;90:4371-5.
33. Baumann U, Preece MA, Green A, Kelly DA,
McKiernan PJ. Hyperinsulinism in tyrosinaemia type I. J Inherit Metab
Dis. 2005;28:131-35.
34. Kapoor RR, Flanagan SE, Arya VB, Shield JP,
Ellard S, Hussain K. Clinical and molecular characterisation of 300
patients with congenital hyperinsulinism. Eur J Endocrino.
2013;168:557-64.
35. Vannucci RC, Vannucci SJ. Hypoglycemic brain
injury. Semin Neonatol. 2001;6:147-55.
36. Chandran S, Rajadurai VS, Alim AH, Hussain K.
Current perspectives on neonatal hypoglycemia, its management, and
cerebral injury risk. Research and Reports in Neonatology. 2015;5:17-30.
37. Mazor-Aronovitch K, Gillis D, Lobel D, Hirsch HJ,
Pinhas-Hamiel O, Modan-Moses D, et al. Long-term
neurodevelopmental outcome in conservatively treated congenital
hyperinsulinism. Eur J Endocrinol. 2007;157:491-7.
38. Wang L, Fan G, Ji X, Sun B, Guo Q. MRI findings
of brain damage due to neonatal hypoglycemia. Zhonghua Fang She Xue Za
Zhi. 2009,43:42-5.
39. Deshpande S, Ward Platt M. The investigation and
management of neonatal hypoglycaemia. Semin Fetal Neonatal Med.
2005;10:351-61.
40. Senniappan S, Arya VB, Hussain K. The molecular
mechanisms, diagnosis and management of congenital hyperinsulinism.
Indian J Endocr Metab. 2013;17:19-30.
41. Harris DL, Battin MR, Weston PJ, Harding JE.
Continuous glucose monitoring in newborn babies at risk of hypoglycemia.
J Pediatr. 2010;157:198-202.
42. Saif M, kapoor A, Kochar IP, Jindal R. Continuous
glucose monitoring system for congenital hyperinsulinemia. Indian
Pediatr. 2013;50:421-2.
43. Hussain K. Investigations for neonatal
hypoglycaemia. Clin Biochem. 2011;44:465-6.
44. Hussain K, Blankenstein O, De Lonlay P,
Christesen HT. Hyperinsulinaemic hypoglycaemia: biochemical basis and
the importance of maintaining normoglycaemia during management. Arch
Dis Child. 2007;92:568-70.
45. Aynsley-Green A, Hussain K, Hall J, Saudubray JM,
Nihoul-Fékété C, De Lonlay-Debeney P, et al. Practical management
of hyperinsulinism in infancy. Arch Dis Child Fetal Neonatal Ed.
2000;82:F98–107.
46. Tas E, Mahmood B, Garibaldi L, Sperling M. Liver
injury may increase the risk of diazoxide toxicity: a case report. Eur j
Pediatr. 2015;174:403-6.
47. Hussain K. Diagnosis and management of
hyperinsulinaemic hypoglycaemia of infancy. Horm Res. 2008;69:2-13.
48. Kim-Hanh Le Quan Sang, Jean-Baptiste Arnoux,
Asmaa Mamoune, Saint-Martin C, Bellanné-Chantelot C, Valayannopoulos,
et al. Successful treatment of congenital hyperinsulinism with
long-acting release octreotide. Eur J Endocrino. 2012;166:333-9.
49. Modan-Moses D, Koren I, Mazor-Aronovitch K,
Pinhas-Hamiel O, Landau H. Treatment of congenital hyperinsulinism with
Lanreotide acetate (Somatuline Autogel). J Clin Endocrinol Metab. 2011;
96:2312-7.
50. Moens K, Berger V, Ahn JM, Van Schravendijk C,
Hruby VJ, Pipeleers D, et al. Assessment of the role of
interstitial glucagon in the acute glucose secretory responsiveness of
in situ pancreatic beta-cells. Diabetes. 2002;51:669-75.
51. Senniappan S, Alexandrescu S, Tatevian N, Shah P,
Arya V, Flanagan S, et al. Sirolimus therapy in infants with
severe hyperinsulinemic hypoglycemia. N Engl J Med. 2014;370:1131-7.
52. Hussain K, Aynsley-Green A. Hyperinsulinaemic
hypoglycaemia in preterm neonates. Arch Dis Child Fetal Neonatal Ed.
2004;89:F65-7.
53. Mohnike K, Blankenstein O, Christesen HT, de
Lonlay J, Hussain K, Koopmans KP, et al. Proposal for a
standardized protocol for 18-F DOPA-PET (PET/CT) in congenital
hyperinsulinism. Horm Res. 2006;66:40-2.
54. Bax KN, van der Zee DC. The laparoscopic approach
towards hyperinsulinism in children. Sem Ped Surg. 2007;16:245-51.
55. Leibowitz G, Glaser B, Higazi AA, Salameh M,
Cerasi E, Landau H. Hyperinsulinemic hypoglycemia of infancy (nesidioblastosis)
in clinical remission: High incidence of diabetes mellitus and
persistent beta-cell dysfunction at long-term follow-up. J Clin
Endocrinol Metab. 1995;80:386-92.
|
|
 |
|